Newfound Cosmic 'Gateway' Funnels Small, Icy Objects to the Inner Solar System
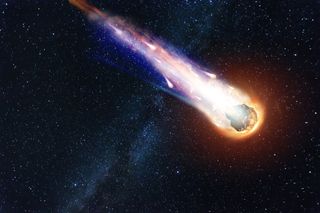
Beginning as small, icy bodies on the outskirts of the solar system , comets turn into spectacular streaks of light when they pass through a "gateway" near Jupiter, according to new research.
This gateway is a region of space where objects called centaurs — small, icy bodies that orbit between Jupiter and Neptune — start to nudge closer to the sun. As they do so, they heat up and become “active,” primarily releasing a dusty halo of gas — which makes these small bodies, technically, comets. "We realized there's a nexus point in orbital space where small bodies change their orbit that we nicknamed 'the gateway,'" said lead author Gal Sarid, a planetary scientist at the University of Central Florida.
The gateway region is like a donut that wraps around the inner solar system, containing many possible orbits within its thick ring. Sarid and his team first came up with the gateway idea after looking at a peculiar centaur named 29P/Schwassmann-Wachmann 1 or SWI. Though technically a centaur, SWI is very active, regularly releasing gases like a comet does, Sarid said.
Related: Fallen Stars: A Gallery of Famous Meteorites
The researchers then modeled other such small, icy bodies in the outskirts of the solar system , and found that many of them took the same path that SWI did — originating beyond Neptune, moving into an erratic orbit between Jupiter and Neptune, and then to this gateway region. In fact, the scientists found that one in five centaurs that they analyzed entered a similar orbit as SW1 at one point in time.
Making this same journey, centaurs give rise to most of the Jupiter family comets, a group of comets called "short-period comets," meaning those that orbit around the sun in less than 200 years. Their models indicate that centaurs don't remain in the gateway region for long; most become Jupiter family comets within a couple of thousand years and move into the inner solar system. That's a short time, considering that comets can live for millions or billions of years.
But this isn't a one-way journey. The researchers' models show that Jupiter family comets also sometimes move back into the gateway region, trekking away from the sun. The data showed that 70% of Jupiter family comets spend time in the gateway region, either moving in toward the inner solar system or moving out to the outskirts. "They can go back and forth like a revolving door," Sarid said.
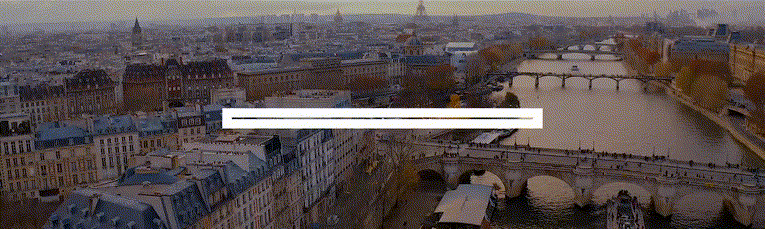
Sign up for the Live Science daily newsletter now
Get the world’s most fascinating discoveries delivered straight to your inbox.
"The gateway model is not meant to solve every possible question," Sarid said. "The idea was to identify this region where this transition happened." This model might eventually tell us a bit about the early solar system, he added. "When the solar system formed very early on it built itself from smaller pieces into bigger pieces," like how you build Ikea furniture, he said. These comets, centaurs and other small objects are like the nuts and bolts of a cabinet.
The findings were first published in the preprint journal arXiv on Aug. 12 and will be published in the Astrophysical Journal Letters this week.
- Photo Gallery: Images of Martian Meteorites
- Space-y Tales: The 5 Strangest Meteorites
- Crash! 10 Biggest Impact Craters on Earth
Originally published on Live Science .
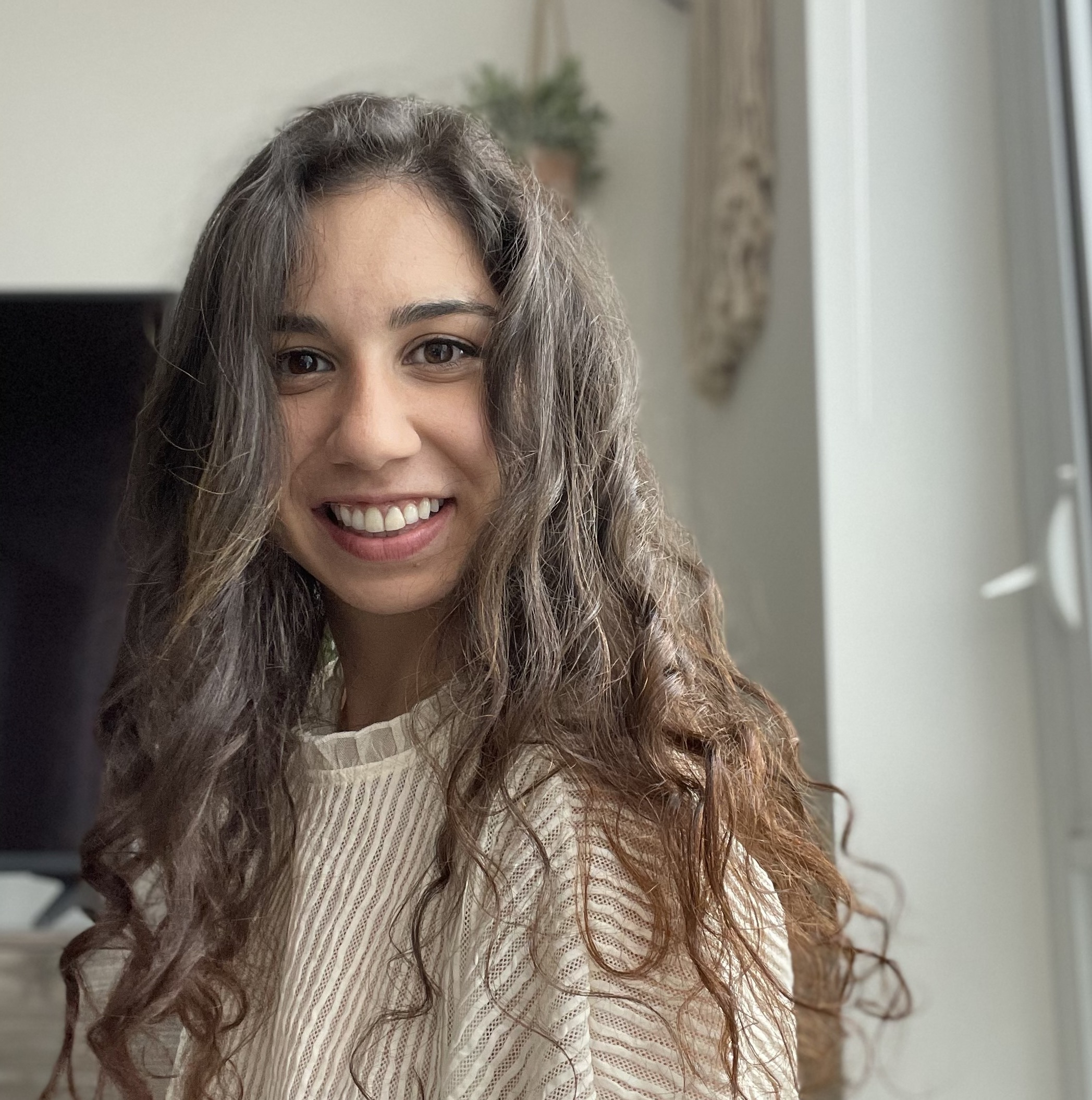
Yasemin is a staff writer at Live Science, covering health, neuroscience and biology. Her work has appeared in Scientific American, Science and the San Jose Mercury News. She has a bachelor's degree in biomedical engineering from the University of Connecticut and a graduate certificate in science communication from the University of California, Santa Cruz.
'Severe' geomagnetic storm will bring widespread auroras this weekend after gigantic sunspot spits out 5 solar storms
Gargantuan sunspot 15-Earths wide shoots powerful X-class flare toward Earth, triggering radio blackouts
Suspected thieves nearly swipe pre-Hispanic artifacts from an archaeological site in Peru
Most Popular
- 2 'Hostilities began in an extremely violent way': How chimp wars taught us murder and cruelty aren't just human traits
- 3 EV batteries could last much longer thanks to new capacitor with 19-times higher energy density that scientists created by mistake
- 4 2,500-year-old Illyrian helmet found in burial mound likely caused 'awe in the enemy'
- 5 Record-shattering Tonga volcanic eruption wasn't triggered by what we thought, new study suggests
- 2 EV batteries could last much longer thanks to new capacitor with 19-times higher energy density that scientists created by mistake
- 3 Roman-era skeletons buried in embrace, on top of a horse, weren't lovers, DNA analysis shows
- 4 30,000 years of history reveals that hard times boost human societies' resilience
- 5 Gargantuan sunspot 15-Earths wide shoots powerful X-class flare toward Earth, triggering radio blackouts
Other Worlds: An Introduction to the Solar System
Overview of our planetary system, learning objectives.
By the end of this section, you will be able to:
- Describe how the objects in our solar system are identified, explored, and characterized
- Describe the types of small bodies in our solar system, their locations, and how they formed
- Model the solar system with distances from everyday life to better comprehend distances in space
The solar system [1] consists of the Sun and many smaller objects: the planets, their moons and rings, and such “debris” as asteroids, comets, and dust. Decades of observation and spacecraft exploration have revealed that most of these objects formed together with the Sun about 4.5 billion years ago. They represent clumps of material that condensed from an enormous cloud of gas and dust. The central part of this cloud became the Sun, and a small fraction of the material in the outer parts eventually formed the other objects.
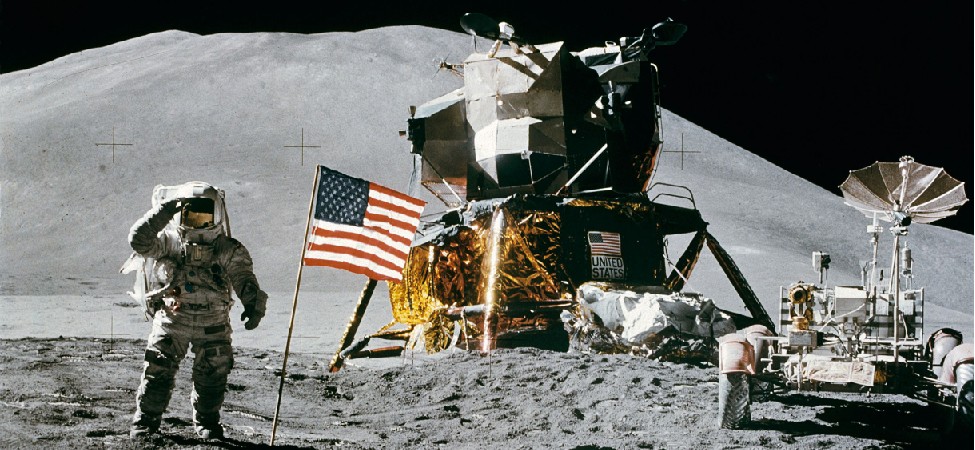
Figure 1: Astronauts on the Moon. The lunar lander and surface rover from the Apollo 15 mission are seen in this view of the one place beyond Earth that has been explored directly by humans. (credit: modification of work by David R. Scott, NASA)
During the past 50 years, we have learned more about the solar system than anyone imagined before the space age. In addition to gathering information with powerful new telescopes, we have sent spacecraft directly to many members of the planetary system. (Planetary astronomy is the only branch of our science in which we can, at least vicariously, travel to the objects we want to study.) With evocative names such as Voyager, Pioneer, Curiosity , and Pathfinder, our robot explorers have flown past, orbited, or landed on every planet, returning images and data that have dazzled both astronomers and the public. In the process, we have also investigated two dwarf planets, hundreds of fascinating moons, four ring systems, a dozen asteroids, and several comets (smaller members of our solar system that we will discuss later).
Our probes have penetrated the atmosphere of Jupiter and landed on the surfaces of Venus, Mars, our Moon , Saturn’s moon Titan, the asteroids Eros and Itokawa, and the Comet Churyumov-Gerasimenko (usually referred to as 67P). Humans have set foot on the Moon and returned samples of its surface soil for laboratory analysis (Figure 1). We have even discovered other places in our solar system that might be able to support some kind of life.
An Inventory
The Sun, a star that is brighter than about 80% of the stars in the Galaxy, is by far the most massive member of the solar system, as shown in Table 1. It is an enormous ball about 1.4 million kilometers in diameter, with surface layers of incandescent gas and an interior temperature of millions of degrees. The Sun will be discussed in later chapters as our first, and best-studied, example of a star.
Table 1 also shows that most of the material of the planets is actually concentrated in the largest one, Jupiter , which is more massive than all the rest of the planets combined. Astronomers were able to determine the masses of the planets centuries ago using Kepler’s laws of planetary motion and Newton’s law of gravity to measure the planets’ gravitational effects on one another or on moons that orbit them (see Orbits and Gravity ). Today, we make even more precise measurements of their masses by tracking their gravitational effects on the motion of spacecraft that pass near them.
Beside Earth, five other planets were known to the ancients—Mercury, Venus, Mars, Jupiter, and Saturn—and two were discovered after the invention of the telescope: Uranus and Neptune. The eight planets all revolve in the same direction around the Sun. They orbit in approximately the same plane, like cars traveling on concentric tracks on a giant, flat racecourse. Each planet stays in its own “traffic lane,” following a nearly circular orbit about the Sun and obeying the “traffic” laws discovered by Galileo, Kepler, and Newton. Besides these planets, we have also been discovering smaller worlds beyond Neptune that are called trans-Neptunian objects or TNOs (see Figure 2). The first to be found, in 1930, was Pluto , but others have been discovered during the twenty-first century. One of them, Eris, is about the same size as Pluto and has at least one moon (Pluto has five known moons.) The largest TNOs are also classed as dwarf planets, as is the largest asteroid, Ceres . (Dwarf planets will be discussed further in the chapter on Rings, Moons, and Pluto ). To date, more than 1750 of these TNOs have been discovered.
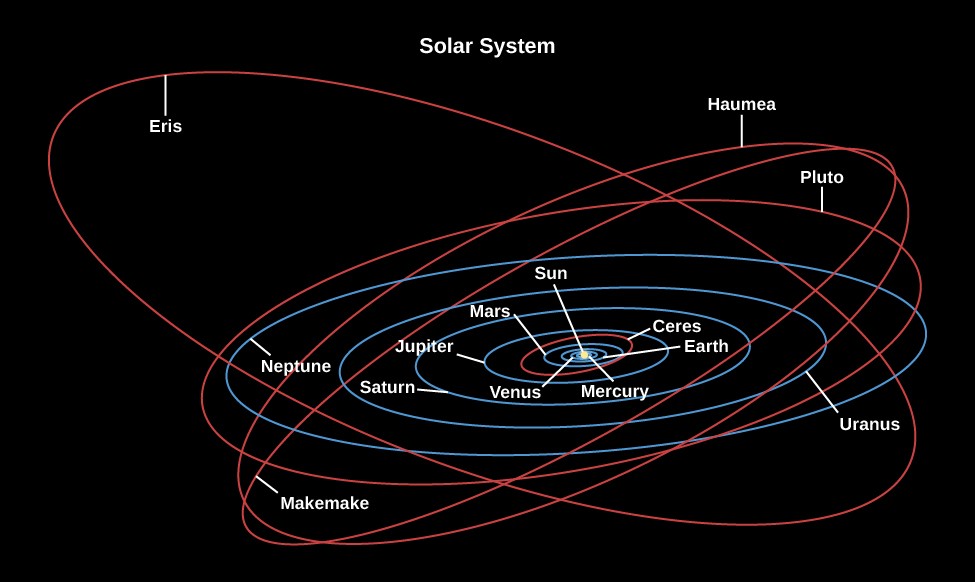
Figure 2: Orbits of the Planets. All eight major planets orbit the Sun in roughly the same plane. The five currently known dwarf planets are also shown: Eris , Haumea , Pluto , Ceres , and Makemake . Note that Pluto’s orbit is not in the plane of the planets.
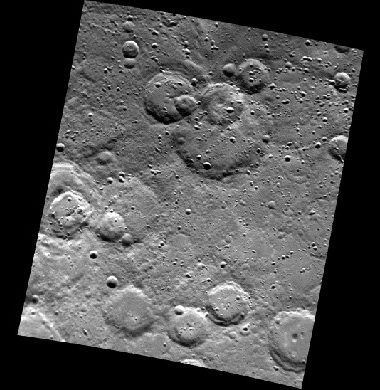
Figure 3: Surface of Mercury. The pockmarked face of the terrestrial world of Mercury is more typical of the inner planets than the watery surface of Earth. This black-and-white image, taken with the Mariner 10 spacecraft, shows a region more than 400 kilometers wide. (credit: modification of work by NASA/John Hopkins University Applied Physics Laboratory/Carnegie Institution of Washington)
Each of the planets and dwarf planets also rotates (spins) about an axis running through it, and in most cases the direction of rotation is the same as the direction of revolution about the Sun. The exceptions are Venus , which rotates backward very slowly (that is, in a retrograde direction), and Uranus and Pluto , which also have strange rotations, each spinning about an axis tipped nearly on its side. We do not yet know the spin orientations of Eris, Haumea, and Makemake.
The four planets closest to the Sun (Mercury through Mars) are called the inner or terrestrial planets . Often, the Moon is also discussed as a part of this group, bringing the total of terrestrial objects to five. (We generally call Earth’s satellite “the Moon,” with a capital M, and the other satellites “moons,” with lowercase m’s.) The terrestrial planets are relatively small worlds, composed primarily of rock and metal. All of them have solid surfaces that bear the records of their geological history in the forms of craters, mountains, and volcanoes (Figure 3).
The next four planets (Jupiter through Neptune) are much larger and are composed primarily of lighter ices, liquids, and gases. We call these four the jovian planets (after “Jove,” another name for Jupiter in mythology) or giant planets —a name they richly deserve (Figure 4). More than 1400 Earths could fit inside Jupiter, for example. These planets do not have solid surfaces on which future explorers might land. They are more like vast, spherical oceans with much smaller, dense cores.
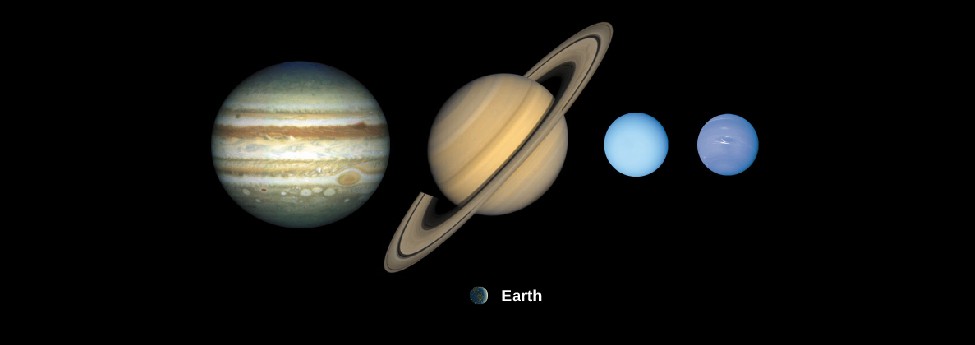
Figure 4: The Four Giant Planets. This montage shows the four giant planets: Jupiter, Saturn, Uranus, and Neptune. Below them, Earth is shown to scale. (credit: modification of work by NASA, Solar System Exploration)
Near the outer edge of the system lies Pluto , which was the first of the distant icy worlds to be discovered beyond Neptune—Pluto was visited by a spacecraft, the NASA New Horizons mission, in 2015 (see Figure 5). Table 2 summarizes some of the main facts about the planets.
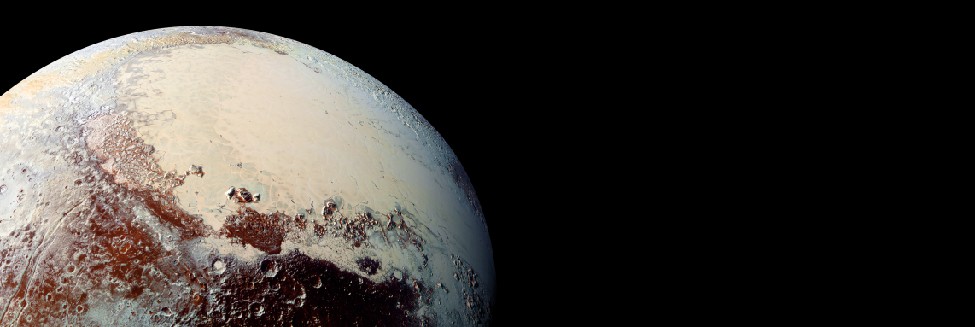
Figure 5: Pluto Close-up. This intriguing image from the New Horizons spacecraft, taken when it flew by the dwarf planet in July 2015, shows some of its complex surface features. The rounded white area is temporarily being called the Sputnik Plain, after humanity’s first spacecraft. (credit: modification of work by NASA/Johns Hopkins University Applied Physics Laboratory/Southwest Research Institute)
Example 1: Comparing Densities
Let’s compare the densities of several members of the solar system. The density of an object equals its mass divided by its volume. The volume ( V ) of a sphere (like a planet) is calculated using the equation
[latex]V=\frac{4}{3}\pi{R}^{3}[/latex]
where π (the Greek letter pi) has a value of approximately 3.14. Although planets are not perfect spheres, this equation works well enough. The masses and diameters of the planets are given in Table 2. For data on selected moons, see Selected Moons of the Planets . Let’s use Saturn’s moon Mimas as our example, with a mass of 4 × 10 19 kg and a diameter of approximately 400 km (radius, 200 km = 2 × 10 5 m).
The volume of Mimas is
[latex]\frac{4}{3}\times 3.14\times {\left(2\times {10}^{5}\text{m}\right)}^{3}=3.3\times {10}^{16}{\text{m}}^{3}[/latex]
Density is mass divided by volume:
[latex]\displaystyle\frac{{4\times 10}^{19}\text{kg}}{{3.3\times 10}^{16}{\text{m}}^{3}}={1.2\times 10}^{3}{\text{kg/m}}^{3}[/latex]
Note that the density of water in these units is 1000 kg/m 3 , so Mimas must be made mainly of ice, not rock. (Note that the density of Mimas given in Selected Moons of the Planets is 1.2, but the units used there are different. In that table, we give density in units of g/cm 3 , for which the density of water equals 1. Can you show, by converting units, that 1 g/cm 3 is the same as 1000 kg/m 3 ?)
Check Your Learning
Calculate the average density of our own planet, Earth. Show your work. How does it compare to the density of an ice moon like Mimas? See Table 2 for data.
For a sphere, [latex]\text{density}=\frac{\text{mass}}{\left(\frac{4}{3}\pi{R}^{3}\right)}{\text{kg/m}}^{3}[/latex]
For Earth, then, [latex]\text{density}=\frac{6\times {10}^{24}\text{kg}}{4.2\times 2.6\times {10}^{20}{\text{m}}^{3}}=5.5\times {10}^{3}{\text{kg/m}}^{3}[/latex]
This density is four to five times greater than Mimas’. In fact, Earth is the densest of the planets.
Smaller Members of the Solar System
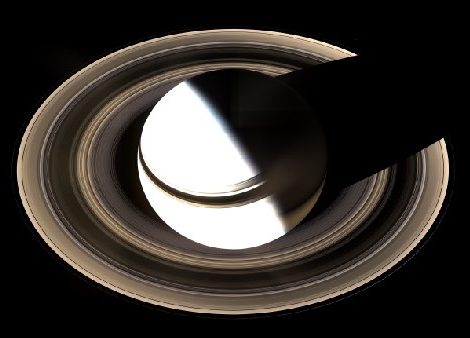
Figure 6: Saturn and Its Rings. This 2007 Cassini image shows Saturn and its complex system of rings, taken from a distance of about 1.2 million kilometers. This natural-color image is a composite of 36 images taken over the course of 2.5 hours. (credit: modification of work by NASA/JPL/Space Science Institute)
Most of the planets are accompanied by one or more moons; only Mercury and Venus move through space alone. There are more than 180 known moons orbiting planets and dwarf planets (see Selected Moons of the Planets for a listing of the larger ones), and undoubtedly many other small ones remain undiscovered.
The largest of the moons are as big as small planets and just as interesting. In addition to our Moon, they include the four largest moons of Jupiter (called the Galilean moons, after their discoverer) and the largest moons of Saturn and Neptune (confusingly named Titan and Triton).
Each of the giant planets also has rings made up of countless small bodies ranging in size from mountains to mere grains of dust, all in orbit about the equator of the planet. The bright rings of Saturn are, by far, the easiest to see. They are among the most beautiful sights in the solar system (Figure 6). But, all four ring systems are interesting to scientists because of their complicated forms, influenced by the pull of the moons that also orbit these giant planets.
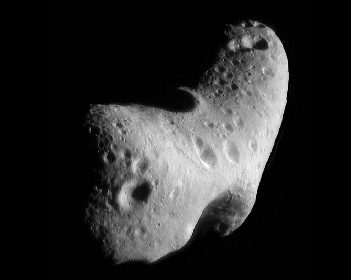
Figure 7: Asteroid Eros. This small Earth-crossing asteroid image was taken by the NEAR-Shoemaker spacecraft from an altitude of about 100 kilometers. This view of the heavily cratered surface is about 10 kilometers wide. The spacecraft orbited Eros for a year before landing gently on its surface. (credit: modification of work by NASA/JHUAPL)
The solar system has many other less-conspicuous members. Another group is the asteroids , rocky bodies that orbit the Sun like miniature planets, mostly in the space between Mars and Jupiter (although some do cross the orbits of planets like Earth—see Figure 7).
Most asteroids are remnants of the initial population of the solar system that existed before the planets themselves formed. Some of the smallest moons of the planets, such as the moons of Mars, are very likely captured asteroids.
Another class of small bodies is composed mostly of ice, made of frozen gases such as water, carbon dioxide, and carbon monoxide; these objects are called comets (see Figure 8).
Comets also are remnants from the formation of the solar system, but they were formed and continue (with rare exceptions) to orbit the Sun in distant, cooler regions—stored in a sort of cosmic deep freeze. This is also the realm of the larger icy worlds, called dwarf planets.
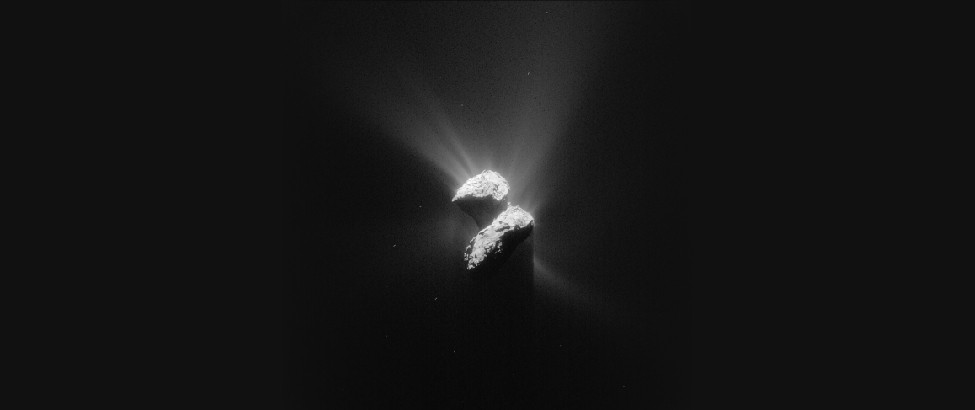
Figure 8: Comet Churyumov-Gerasimenko (67P). This image shows Comet Churyumov-Gerasimenko, also known as 67P, near its closest approach to the Sun in 2015, as seen from the Rosetta spacecraft. Note the jets of gas escaping from the solid surface. (credit: modification of work by ESA/Rosetta/NAVACAM, CC BY-SA IGO 3.0 )
Finally, there are countless grains of broken rock, which we call cosmic dust, scattered throughout the solar system. When these particles enter Earth’s atmosphere (as millions do each day) they burn up, producing a brief flash of light in the night sky known as a meteor (meteors are often referred to as shooting stars). Occasionally, some larger chunk of rocky or metallic material survives its passage through the atmosphere and lands on Earth. Any piece that strikes the ground is known as a meteorite. (You can see meteorites on display in many natural history museums and can sometimes even purchase pieces of them from gem and mineral dealers.)
Carl Sagan: Solar System Advocate
The best-known astronomer in the world during the 1970s and 1980s, Carl Sagan devoted most of his professional career to studying the planets and considerable energy to raising public awareness of what we can learn from exploring the solar system (see Figure 9). Born in Brooklyn, New York, in 1934, Sagan became interested in astronomy as a youngster; he also credits science fiction stories for sustaining his fascination with what’s out in the universe.
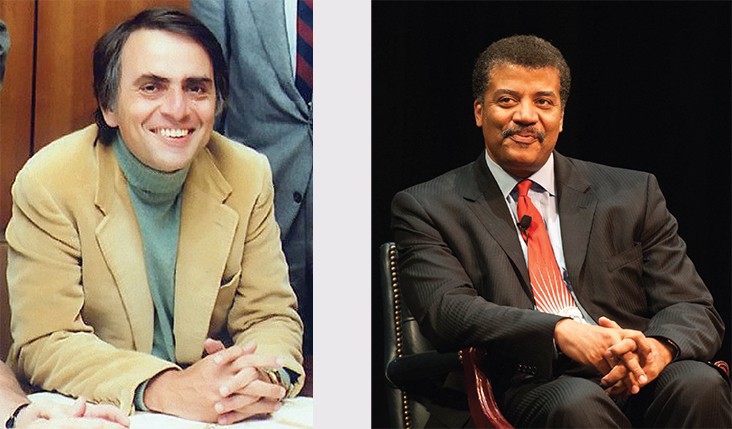
Figure 9: Carl Sagan (1934–1996) and Neil deGrasse Tyson. Sagan was Tyson’s inspiration to become a scientist. (credit “Sagan”: modification of work by NASA, JPL; credit “Tyson”: modification of work by Bruce F. Press)
In the early 1960s, when many scientists still thought Venus might turn out to be a hospitable place, Sagan calculated that the thick atmosphere of Venus could act like a giant greenhouse, keeping the heat in and raising the temperature enormously. He showed that the seasonal changes astronomers had seen on Mars were caused, not by vegetation, but by wind-blown dust. He was a member of the scientific teams for many of the robotic missions that explored the solar system and was instrumental in getting NASA to put a message-bearing plaque aboard the Pioneer spacecraft, as well as audio-video records on the Voyager spacecraft—all of them destined to leave our solar system entirely and send these little bits of Earth technology out among the stars.
To encourage public interest and public support of planetary exploration, Sagan helped found The Planetary Society, now the largest space-interest organization in the world. He was a tireless and eloquent advocate of the need to study the solar system close-up and the value of learning about other worlds in order to take better care of our own.
Sagan simulated conditions on early Earth to demonstrate how some of life’s fundamental building blocks might have formed from the “primordial soup” of natural compounds on our planet. In addition, he and his colleagues developed computer models showing the consequences of nuclear war for Earth would be even more devastating than anyone had thought (this is now called the nuclear winter hypothesis) and demonstrating some of the serious consequences of continued pollution of our atmosphere.
Sagan was perhaps best known, however, as a brilliant popularizer of astronomy and the author of many books on science, including the best-selling Cosmos , and several evocative tributes to solar system exploration such as The Cosmic Connection and Pale Blue Dot . His book The Demon Haunted World , completed just before his death in 1996, is perhaps the best antidote to fuzzy thinking about pseudo-science and irrationality in print today. An intriguing science fiction novel he wrote, titled Contact , which became a successful film as well, is still recommended by many science instructors as a scenario for making contact with life elsewhere that is much more reasonable than most science fiction.
Sagan was a master, too, of the television medium. His 13-part public television series, Cosmos , was seen by an estimated 500 million people in 60 countries and has become one of the most-watched series in the history of public broadcasting. A few astronomers scoffed at a scientist who spent so much time in the public eye, but it is probably fair to say that Sagan’s enthusiasm and skill as an explainer won more friends for the science of astronomy than anyone or anything else in the second half of the twentieth century.
In the two decades since Sagan’s death, no other scientist has achieved the same level of public recognition. Perhaps closest is the director of the Hayden Planetarium, Neil deGrasse Tyson, who followed in Sagan’s footsteps by making an updated version of the Cosmos program in 2014. Tyson is quick to point out that Sagan was his inspiration to become a scientist, telling how Sagan invited him to visit for a day at Cornell when he was a high school student looking for a career. However, the media environment has fragmented a great deal since Sagan’s time. It is interesting to speculate whether Sagan could have adapted his communication style to the world of cable television, Twitter, Facebook, and podcasts.
Two imaginative videos provide a tour of the solar system objects we have been discussing. Shane Gellert’s I Need Some Space uses NASA photography and models to show the various worlds with which we share our system:
In the more science fiction-oriented Wanderers video, we see some of the planets and moons as tourist destinations for future explorers, with commentary taken from recordings by Carl Sagan:
A Scale Model of the Solar System
Astronomy often deals with dimensions and distances that far exceed our ordinary experience. What does 1.4 billion kilometers—the distance from the Sun to Saturn—really mean to anyone? It can be helpful to visualize such large systems in terms of a scale model.
In our imaginations, let us build a scale model of the solar system, adopting a scale factor of 1 billion (10 9 )—that is, reducing the actual solar system by dividing every dimension by a factor of 10 9 . Earth, then, has a diameter of 1.3 centimeters, about the size of a grape. The Moon is a pea orbiting this at a distance of 40 centimeters, or a little more than a foot away. The Earth-Moon system fits into a standard backpack.
In this model, the Sun is nearly 1.5 meters in diameter, about the average height of an adult, and our Earth is at a distance of 150 meters—about one city block—from the Sun. Jupiter is five blocks away from the Sun, and its diameter is 15 centimeters, about the size of a very large grapefruit. Saturn is 10 blocks from the Sun; Uranus, 20 blocks; and Neptune, 30 blocks. Pluto, with a distance that varies quite a bit during its 249-year orbit, is currently just beyond 30 blocks and getting farther with time. Most of the moons of the outer solar system are the sizes of various kinds of seeds orbiting the grapefruit, oranges, and lemons that represent the outer planets.
In our scale model, a human is reduced to the dimensions of a single atom, and cars and spacecraft to the size of molecules. Sending the Voyager spacecraft to Neptune involves navigating a single molecule from the Earth–grape toward a lemon 5 kilometers away with an accuracy equivalent to the width of a thread in a spider’s web.
If that model represents the solar system, where would the nearest stars be? If we keep the same scale, the closest stars would be tens of thousands of kilometers away. If you built this scale model in the city where you live, you would have to place the representations of these stars on the other side of Earth or beyond.
By the way, model solar systems like the one we just presented have been built in cities throughout the world. In Sweden, for example, Stockholm’s huge Globe Arena has become a model for the Sun, and Pluto is represented by a 12-centimeter sculpture in the small town of Delsbo, 300 kilometers away. Another model solar system is in Washington on the Mall between the White House and Congress (perhaps proving they are worlds apart?).
Names in the Solar System
We humans just don’t feel comfortable until something has a name. Types of butterflies, new elements, and the mountains of Venus all need names for us to feel we are acquainted with them. How do we give names to objects and features in the solar system?
Planets and moons are named after gods and heroes in Greek and Roman mythology (with a few exceptions among the moons of Uranus, which have names drawn from English literature). When William Herschel, a German immigrant to England, first discovered the planet we now call Uranus, he wanted to name it Georgium Sidus (George’s star) after King George III of his adopted country. This caused such an outcry among astronomers in other nations, however, that the classic tradition was upheld—and has been maintained ever since. Luckily, there were a lot of minor gods in the ancient pantheon, so plenty of names are left for the many small moons we are discovering around the giant planets. ( Selected Moons of the Planets lists the larger moons).
Comets are often named after their discoverers (offering an extra incentive to comet hunters). Asteroids are named by their discoverers after just about anyone or anything they want. Recently, asteroid names have been used to recognize people who have made significant contributions to astronomy, including the three original authors of this book.
That was pretty much all the naming that was needed while our study of the solar system was confined to Earth. But now, our spacecraft have surveyed and photographed many worlds in great detail, and each world has a host of features that also need names. To make sure that naming things in space remains multinational, rational, and somewhat dignified, astronomers have given the responsibility of approving names to a special committee of the International Astronomical Union (IAU), the body that includes scientists from every country that does astronomy.
This IAU committee has developed a set of rules for naming features on other worlds. For example, craters on Venus are named for women who have made significant contributions to human knowledge and welfare. Volcanic features on Jupiter’s moon Io, which is in a constant state of volcanic activity, are named after gods of fire and thunder from the mythologies of many cultures. Craters on Mercury commemorate famous novelists, playwrights, artists, and composers. On Saturn’s moon Tethys, all the features are named after characters and places in Homer’s great epic poem, The Odyssey . As we explore further, it may well turn out that more places in the solar system need names than Earth history can provide. Perhaps by then, explorers and settlers on these worlds will be ready to develop their own names for the places they may (if but for a while) call home.
You may be surprised to know that the meaning of the word planet has recently become controversial because we have discovered many other planetary systems that don’t look very much like our own. Even within our solar system, the planets differ greatly in size and chemical properties. The biggest dispute concerns Pluto, which is much smaller than the other eight major planets. The category of dwarf planet was invented to include Pluto and similar icy objects beyond Neptune. But is a dwarf planet also a planet? Logically, it should be, but even this simple issue of grammar has been the subject of heated debate among both astronomers and the general public.
Key Concepts and Summary
Our solar system currently consists of the Sun, eight planets, five dwarf planets, nearly 200 known moons, and a host of smaller objects. The planets can be divided into two groups: the inner terrestrial planets and the outer giant planets. Pluto, Eris, Haumea, and Makemake do not fit into either category; as icy dwarf planets, they exist in an ice realm on the fringes of the main planetary system. The giant planets are composed mostly of liquids and gases. Smaller members of the solar system include asteroids (including the dwarf planet Ceres), which are rocky and metallic objects found mostly between Mars and Jupiter; comets, which are made mostly of frozen gases and generally orbit far from the Sun; and countless smaller grains of cosmic dust. When a meteor survives its passage through our atmosphere and falls to Earth, we call it a meteorite.
asteroid: a stony or metallic object orbiting the Sun that is smaller than a major planet but that shows no evidence of an atmosphere or of other types of activity associated with comets
comet: a small body of icy and dusty matter that revolves about the Sun; when a comet comes near the Sun, some of its material vaporizes, forming a large head of tenuous gas and often a tail
giant planet: any of the planets Jupiter, Saturn, Uranus, and Neptune in our solar system, or planets of roughly that mass and composition in other planetary systems
meteor: a small piece of solid matter that enters Earth’s atmosphere and burns up, popularly called a shooting star because it is seen as a small flash of light
meteorite: a portion of a meteor that survives passage through an atmosphere and strikes the ground
terrestrial planet: any of the planets Mercury, Venus, Earth, or Mars; sometimes the Moon is included in the list
- The generic term for a group of planets and other bodies circling a star is planetary system . Ours is called the solar system because our Sun is sometimes called Sol . Strictly speaking, then, there is only one solar system; planets orbiting other stars are in planetary systems. ↵
- An AU (or astronomical unit) is the distance from Earth to the Sun. ↵
- We give densities in units where the density of water is 1 g/cm 3 . To get densities in units of kg/m 3 , multiply the given value by 1000. ↵
- Astronomy. Provided by : OpenStax CNX. Located at : http://cnx.org/contents/[email protected] . License : CC BY: Attribution . License Terms : Download for free at http://cnx.org/contents/[email protected].
- I Need Some Space. Authored by : BlueDogFilms. Located at : https://vimeo.com/78449289 . License : All Rights Reserved
- Wanderers. Authored by : Erik Wernquist. Located at : https://vimeo.com/108650530 . License : All Rights Reserved
7.1 Overview of Our Planetary System
Learning objectives.
By the end of this section, you will be able to:
- Describe how the objects in our solar system are identified, explored, and characterized
- Describe the types of small bodies in our solar system, their locations, and how they formed
- Model the solar system with distances from everyday life to better comprehend distances in space
The solar system 1 consists of the Sun and many smaller objects: the planets, their moons and rings, and such “debris” as asteroids, comets, and dust. Decades of observation and spacecraft exploration have revealed that most of these objects formed together with the Sun about 4.5 billion years ago. They represent clumps of material that condensed from an enormous cloud of gas and dust. The central part of this cloud became the Sun, and a small fraction of the material in the outer parts eventually formed the other objects.
During the past 50 years, we have learned more about the solar system than anyone imagined before the space age. In addition to gathering information with powerful new telescopes, we have sent spacecraft directly to many members of the planetary system. (Planetary astronomy is the only branch of our science in which we can, at least vicariously, travel to the objects we want to study.) With evocative names such as Voyager , Pioneer , Curiosity , and Pathfinder , our robot explorers have flown past, orbited, or landed on every planet, returning images and data that have dazzled both astronomers and the public. In the process, we have also investigated two dwarf planets, hundreds of fascinating moons, four ring systems, a dozen asteroids, and several comets (smaller members of our solar system that we will discuss later).
Our probes have penetrated the atmosphere of Jupiter and landed on the surfaces of Venus, Mars, our Moon , Saturn’s moon Titan, the asteroids Eros, Itokawa, Ryugu, and Bennu, and the Comet Churyumov-Gerasimenko (usually referred to as 67P). Humans have set foot on the Moon and returned samples of its surface soil for laboratory analysis ( Figure 7.2 ). We have flown a helicopter drone on Mars. We have even discovered other places in our solar system that might be able to support some kind of life.
Link to Learning
View this gallery of NASA images that trace the history of the Apollo mission.
An Inventory
The Sun, a star that is brighter than about 80% of the stars in the Galaxy, is by far the most massive member of the solar system, as shown in Table 7.1 . It is an enormous ball about 1.4 million kilometers in diameter, with surface layers of incandescent gas and an interior temperature of millions of degrees. The Sun will be discussed in later chapters as our first, and best-studied, example of a star.
Table 7.1 also shows that most of the material of the planets is actually concentrated in the largest one, Jupiter , which is more massive than all the rest of the planets combined. Astronomers were able to determine the masses of the planets centuries ago using Kepler’s laws of planetary motion and Newton’s law of gravity to measure the planets’ gravitational effects on one another or on moons that orbit them (see Orbits and Gravity ). Today, we make even more precise measurements of their masses by tracking their gravitational effects on the motion of spacecraft that pass near them.
Beside Earth, five other planets were known to the ancients—Mercury, Venus, Mars, Jupiter, and Saturn—and two were discovered after the invention of the telescope: Uranus and Neptune. The eight planets all revolve in the same direction around the Sun. They orbit in approximately the same plane, like cars traveling on concentric tracks on a giant, flat racecourse. Each planet stays in its own “traffic lane,” following a nearly circular orbit about the Sun and obeying the “traffic” laws discovered by Galileo, Kepler, and Newton. Besides these planets, we have also been discovering smaller worlds beyond Neptune that are called trans-Neptunian object s or TNOs (see Figure 7.3 ). The first to be found, in 1930, was Pluto , but others have been discovered during the twenty-first century. One of them, Eris , is about the same size as Pluto and has at least one moon (Pluto has five known moons.) The largest TNOs are also classed as dwarf planets, as is the largest asteroid, Ceres . (Dwarf planets will be discussed further in the chapter on Rings, Moons, and Pluto ). To date, more than 2600 of these TNOs have been discovered, and one, called Arrokoth, was explored by the New Horizons spacecraft.
Each of the planets and dwarf planets also rotates (spins) about an axis running through it, and in most cases the direction of rotation is the same as the direction of revolution about the Sun. The exceptions are Venus , which rotates backward very slowly (that is, in a retrograde direction), and Uranus and Pluto , which also have strange rotations, each spinning about an axis tipped nearly on its side. We do not yet know the spin orientations of Eris, Haumea, and Makemake.
The four planets closest to the Sun (Mercury through Mars) are called the inner or terrestrial planets . Often, the Moon is also discussed as a part of this group, bringing the total of terrestrial objects to five. (We generally call Earth’s satellite “the Moon,” with a capital M, and the other satellites “moons,” with lowercase m’s.) The terrestrial planets are relatively small worlds, composed primarily of rock and metal. All of them have solid surfaces that bear the records of their geological history in the forms of craters, mountains, and volcanoes ( Figure 7.4 ).
The next four planets (Jupiter through Neptune) are much larger and are composed primarily of lighter ices, liquids, and gases. We call these four the jovian planets (after “Jove,” another name for Jupiter in mythology) or giant planets —a name they richly deserve ( Figure 7.5 ). About 1,300 Earths could fit inside Jupiter, for example. These planets do not have solid surfaces on which future explorers might land. They are more like vast, spherical oceans with much smaller, dense cores.
Near the outer edge of the system lies Pluto , which was the first of the distant icy worlds to be discovered beyond Neptune (Pluto was visited by a spacecraft, the NASA New Horizons mission, in 2015 [see Figure 7.6 ]). Table 7.2 summarizes some of the main facts about the planets.
Example 7.1
Comparing densities.
where π (the Greek letter pi) has a value of approximately 3.14. Although planets are not perfect spheres, this equation works well enough. The masses and diameters of the planets are given in Table 7.2 . For data on selected moons, see Appendix G . Let’s use Saturn’s moon Mimas as our example, with a mass of 4 × 10 19 kg and a diameter of approximately 400 km (radius, 200 km = 2 × 10 5 m).
Density is mass divided by volume:
Note that the density of water in these units is 1000 kg/m 3 , so Mimas must be made mainly of ice, not rock. (Note that the density of Mimas given in Appendix G is 1.2, but the units used there are different. In that table, we give density in units of g/cm 3 , for which the density of water equals 1. Can you show, by converting units, that 1 g/cm 3 is the same as 1000 kg/m 3 ?)
Check Your Learning
For a sphere, density = mass ( 4 3 π R 3 ) kg/m 3 . density = mass ( 4 3 π R 3 ) kg/m 3 . For Earth, then, density = 6 × 10 24 kg 4.2 × 2.6 × 10 20 m 3 = 5.5 × 10 3 kg/m 3 . density = 6 × 10 24 kg 4.2 × 2.6 × 10 20 m 3 = 5.5 × 10 3 kg/m 3 . This density is four to five times greater than Mimas’. In fact, Earth is the densest of the planets.
Learn more about NASA’s mission to Pluto and see high-resolution images of Pluto and its moon Charon.
Smaller Members of the Solar System
Most of the planets are accompanied by one or more moons; only Mercury and Venus move through space alone. There are more than 210 known moons orbiting planets and dwarf planets (see Appendix G for a listing of the larger ones), and undoubtedly many other small ones remain undiscovered. The largest of the moons are as big as small planets and just as interesting. In addition to our Moon, they include the four largest moons of Jupiter (called the Galilean moons, after their discoverer) and the largest moons of Saturn and Neptune (confusingly named Titan and Triton).
Each of the giant planets also has rings made up of countless small bodies ranging in size from mountains to mere grains of dust, all in orbit about the equator of the planet. The bright rings of Saturn are, by far, the easiest to see. They are among the most beautiful sights in the solar system ( Figure 7.7 ). But, all four ring systems are interesting to scientists because of their complicated forms, influenced by the pull of the moons that also orbit these giant planets.
The solar system has many other less-conspicuous members. Another group is the asteroids , rocky bodies that orbit the Sun like miniature planets, mostly in the space between Mars and Jupiter (although some do cross the orbits of planets like Earth—see Figure 7.8 ). Most asteroids are remnants of the initial population of the solar system that existed before the planets themselves formed. Some of the smallest moons of the planets, such as the moons of Mars, are very likely captured asteroids.
Another class of small bodies is composed mostly of ice, made of frozen gases such as water, carbon dioxide, and carbon monoxide; these objects are called comets (see Figure 7.9 ). Comets also are remnants from the formation of the solar system, but they were formed and continue (with rare exceptions) to orbit the Sun in distant, cooler regions—stored in a sort of cosmic deep freeze. This is also the realm of the larger icy worlds, called dwarf planets.
Finally, there are countless grains of broken rock, which we call cosmic dust, scattered throughout the solar system. When these particles enter Earth’s atmosphere (as millions do each day) they burn up, producing a brief flash of light in the night sky known as a meteor (meteors are often referred to as shooting stars). Occasionally, some larger chunk of rocky or metallic material survives its passage through the atmosphere and lands on Earth. Any piece that strikes the ground is known as a meteorite . (You can see meteorites on display in many natural history museums and can sometimes even purchase pieces of them from gem and mineral dealers.)
Voyagers in Astronomy
Carl sagan: solar system advocate.
The best-known astronomer in the world during the 1970s and 1980s, Carl Sagan devoted most of his professional career to studying the planets and considerable energy to raising public awareness of what we can learn from exploring the solar system (see Figure 7.10 ). Born in Brooklyn, New York, in 1934, Sagan became interested in astronomy as a youngster; he also credits science fiction stories for sustaining his fascination with what’s out in the universe.
In the early 1960s, when many scientists still thought Venus might turn out to be a hospitable place, Sagan calculated that the thick atmosphere of Venus could act like a giant greenhouse, keeping the heat in and raising the temperature enormously. He showed that the seasonal changes astronomers had seen on Mars were caused, not by vegetation, but by wind-blown dust. He was a member of the scientific teams for many of the robotic missions that explored the solar system and was instrumental in getting NASA to put a message-bearing plaque aboard the Pioneer spacecraft, as well as audio-video records on the Voyager spacecraft—all of them destined to leave our solar system entirely and send these little bits of Earth technology out among the stars.
To encourage public interest and public support of planetary exploration, Sagan helped found The Planetary Society, now the largest space-interest organization in the world. He was a tireless and eloquent advocate of the need to study the solar system close-up and the value of learning about other worlds in order to take better care of our own.
Sagan simulated conditions on early Earth to demonstrate how some of life’s fundamental building blocks might have formed from the “primordial soup” of natural compounds on our planet. In addition, he and his colleagues developed computer models showing the consequences of nuclear war for Earth would be even more devastating than anyone had thought (this is now called the nuclear winter hypothesis) and demonstrating some of the serious consequences of continued pollution of our atmosphere.
Sagan was perhaps best known, however, as a brilliant popularizer of astronomy and the author of many books on science, including the best-selling Cosmos , and several evocative tributes to solar system exploration such as The Cosmic Connection and Pale Blue Dot . His book The Demon Haunted World , completed just before his death in 1996, is perhaps the best antidote to fuzzy thinking about pseudo-science and irrationality in print today. An intriguing science fiction novel he wrote, titled Contact , which became a successful film as well, is still recommended by many science instructors as a scenario for making contact with life elsewhere that is much more reasonable than most science fiction.
Sagan was a master, too, of the television medium. His 13-part public television series, Cosmos , was seen by an estimated 500 million people in 60 countries and has become one of the most-watched series in the history of public broadcasting. A few astronomers scoffed at a scientist who spent so much time in the public eye, but it is probably fair to say that Sagan’s enthusiasm and skill as an explainer won more friends for the science of astronomy than anyone or anything else in the second half of the twentieth century.
In the two decades since Sagan’s death, no other scientist has achieved the same level of public recognition. Perhaps closest is the director of the Hayden Planetarium, Neil deGrasse Tyson , who followed in Sagan’s footsteps by making an updated version of the Cosmos program in 2014. Tyson is quick to point out that Sagan was his inspiration to become a scientist, telling how Sagan invited him to visit for a day at Cornell when he was a high school student looking for a career. However, the media environment has fragmented a great deal since Sagan’s time. It is interesting to speculate whether Sagan could have adapted his communication style to the world of cable television, Twitter, Facebook, and podcasts.
Two imaginative videos provide a tour of the solar system objects we have been discussing. Shane Gellert’s I Need Some Space uses NASA photography and models to show the various worlds with which we share our system. In the more science fiction-oriented Wanderers video, we see some of the planets and moons as tourist destinations for future explorers, with commentary taken from recordings by Carl Sagan.
A Scale Model of the Solar System
Astronomy often deals with dimensions and distances that far exceed our ordinary experience. What does 1.4 billion kilometers—the distance from the Sun to Saturn—really mean to anyone? It can be helpful to visualize such large systems in terms of a scale model.
In our imaginations, let us build a scale model of the solar system, adopting a scale factor of 1 billion (10 9 )—that is, reducing the actual solar system by dividing every dimension by a factor of 10 9 . Earth, then, has a diameter of 1.3 centimeters, about the size of a grape. The Moon is a pea orbiting this at a distance of 40 centimeters, or a little more than a foot away. The Earth-Moon system fits into a standard backpack.
In this model, the Sun is nearly 1.5 meters in diameter, about the average height of an adult, and our Earth is at a distance of 150 meters—about one city block—from the Sun. Jupiter is five blocks away from the Sun, and its diameter is 15 centimeters, about the size of a very large grapefruit. Saturn is 10 blocks from the Sun; Uranus, 20 blocks; and Neptune, 30 blocks. Pluto, with a distance that varies quite a bit during its 249-year orbit, is currently just beyond 30 blocks and getting farther with time. Most of the moons of the outer solar system are the sizes of various kinds of seeds orbiting the grapefruit, oranges, and lemons that represent the outer planets.
In our scale model, a human is reduced to the dimensions of a single atom, and cars and spacecraft to the size of molecules. Sending the Voyager spacecraft to Neptune involves navigating a single molecule from the Earth–grape toward a lemon 5 kilometers away with an accuracy equivalent to the width of a thread in a spider’s web.
If that model represents the solar system, where would the nearest stars be? If we keep the same scale, the closest stars would be tens of thousands of kilometers away. If you built this scale model in the city where you live, you would have to place the representations of these stars on the other side of Earth or beyond.
By the way, model solar systems like the one we just presented have been built in cities throughout the world. In Sweden, for example, Stockholm’s huge Globe Arena has become a model for the Sun, and Pluto is represented by a 12-centimeter sculpture in the small town of Delsbo, 300 kilometers away. Another model solar system is in Washington on the Mall between the White House and Congress (perhaps proving they are worlds apart?).
This model of the solar system shows all orbits and sizes to scale, and it lets you fly between the planets at an enhanced speed.
Making Connections
Names in the solar system.
We humans just don’t feel comfortable until something has a name. Types of butterflies, new elements, and the mountains of Venus all need names for us to feel we are acquainted with them. How do we give names to objects and features in the solar system?
Planets and moons are named after gods and heroes in Greek and Roman mythology (with a few exceptions among the moons of Uranus, which have names drawn from English literature). When William Herschel, a German immigrant to England, first discovered the planet we now call Uranus, he wanted to name it Georgium Sidus (George’s star) after King George III of his adopted country. This caused such an outcry among astronomers in other nations, however, that the classic tradition was upheld—and has been maintained ever since. Luckily, there were a lot of minor gods in the ancient pantheon, so plenty of names are left for the many small moons we are discovering around the giant planets. ( Appendix G lists the larger moons). More recently, the names of dwarf planets and their moons have been drawn from the mythology of other cultures besides Greek and Roman.
Comets are often named after their discoverers (offering an extra incentive to comet hunters). Asteroids are named by their discoverers after just about anyone or anything they want. Recently, asteroid names have been used to recognize people who have made significant contributions to astronomy, including the three senior authors of this book.
That was pretty much all the naming that was needed while our study of the solar system was confined to Earth. But now, our spacecraft have surveyed and photographed many worlds in great detail, and each world has a host of features that also need names. To make sure that naming things in space remains multinational, rational, and somewhat dignified, astronomers have given the responsibility of approving names to a special committee of the International Astronomical Union (IAU), the body that includes scientists from every country that does astronomy.
This IAU committee has developed a set of rules for naming features on other worlds. For example, craters on Venus are named for women who have made significant contributions to human knowledge and welfare. Volcanic features on Jupiter’s moon Io, which is in a constant state of volcanic activity, are named after gods of fire and thunder from the mythologies of many cultures. Craters on Mercury commemorate famous novelists, playwrights, artists, and composers. On Saturn’s moon Tethys, all the features are named after characters and places in Homer’s great epic poem, The Odyssey . As we explore further, it may well turn out that more places in the solar system need names than Earth history can provide. Perhaps by then, explorers and settlers on these worlds will be ready to develop their own names for the places they may (if but for a while) call home.
You may be surprised to know that the meaning of the word planet has recently become controversial because we have discovered many other planetary systems that don’t look very much like our own. Even within our solar system, the planets differ greatly in size and chemical properties. The biggest dispute concerns Pluto, which is much smaller than the other eight major planets. The category of dwarf planet was invented to include Pluto and similar icy objects beyond Neptune. But is a dwarf planet also a planet? Logically, it should be, but even this simple issue of grammar has been the subject of heated debate among both astronomers and the general public.
- 1 The generic term for a group of planets and other bodies circling a star is planetary system . Ours is called the solar system because our Sun is sometimes called Sol . Strictly speaking, then, there is only one solar system; planets orbiting other stars are in planetary systems.
- 2 An AU (or astronomical unit) is the distance from Earth to the Sun.
- 3 We give densities in units where the density of water is 1 g/cm 3 . To get densities in units of kg/m 3 , multiply the given value by 1000.
As an Amazon Associate we earn from qualifying purchases.
This book may not be used in the training of large language models or otherwise be ingested into large language models or generative AI offerings without OpenStax's permission.
Want to cite, share, or modify this book? This book uses the Creative Commons Attribution License and you must attribute OpenStax.
Access for free at https://openstax.org/books/astronomy/pages/1-introduction
- Authors: Andrew Fraknoi, David Morrison, Sidney C. Wolff
- Publisher/website: OpenStax
- Book title: Astronomy
- Publication date: Oct 13, 2016
- Location: Houston, Texas
- Book URL: https://openstax.org/books/astronomy/pages/1-introduction
- Section URL: https://openstax.org/books/astronomy/pages/7-1-overview-of-our-planetary-system
© Jan 28, 2022 OpenStax. Textbook content produced by OpenStax is licensed under a Creative Commons Attribution License . The OpenStax name, OpenStax logo, OpenStax book covers, OpenStax CNX name, and OpenStax CNX logo are not subject to the Creative Commons license and may not be reproduced without the prior and express written consent of Rice University.
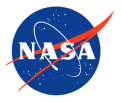
Suggested Searches
- Climate Change
- Expedition 64
- Mars perseverance
- SpaceX Crew-2
- International Space Station
- View All Topics A-Z
- Humans in Space
Earth & Climate
The solar system, the universe, aeronautics, learning resources, news & events.
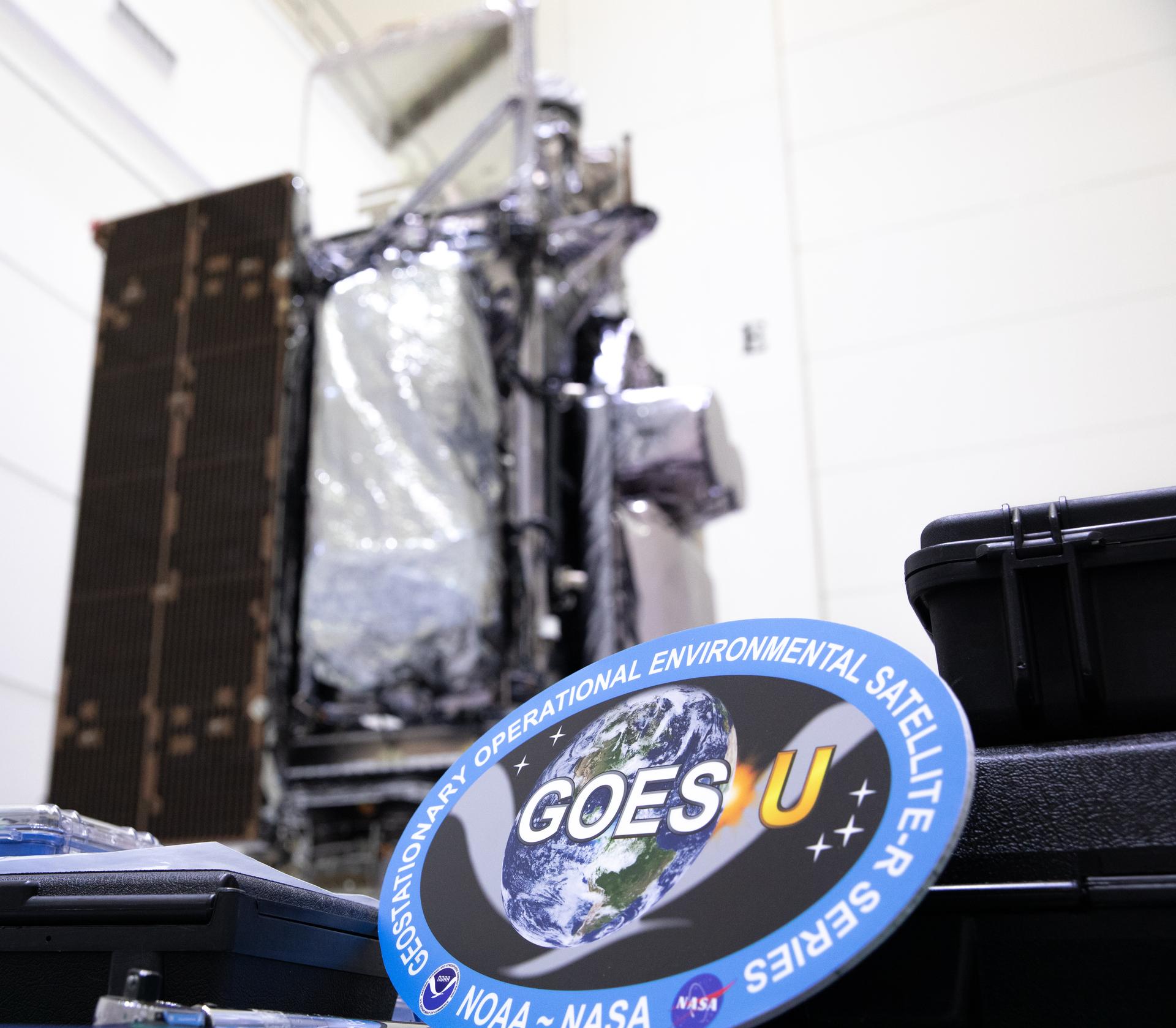
NASA Invites Social Creators for Launch of NOAA Weather Satellite
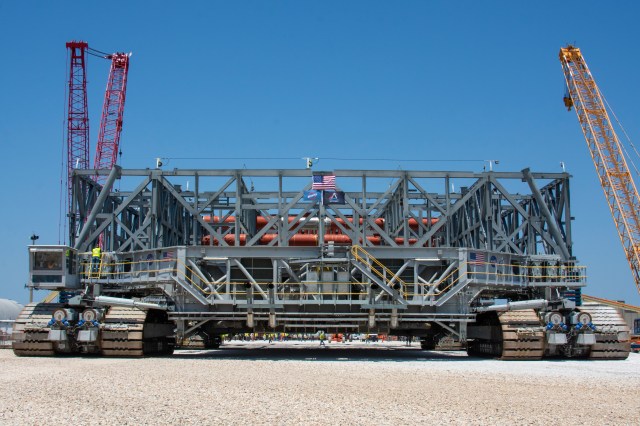
NASA’s New Mobile Launcher Stacks Up for Future Artemis Missions
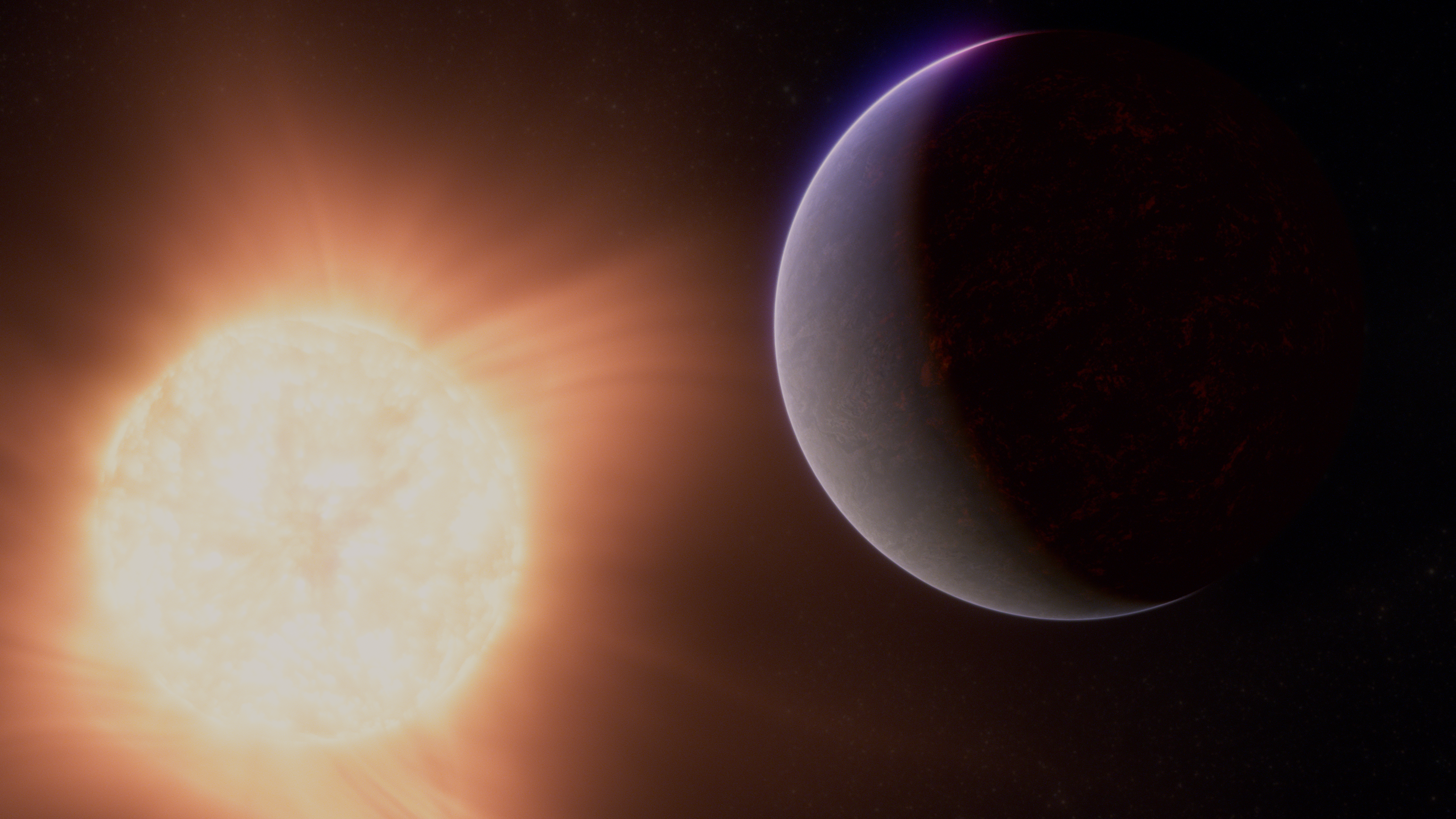
NASA’s Webb Hints at Possible Atmosphere Surrounding Rocky Exoplanet
- Search All NASA Missions
- A to Z List of Missions
- Upcoming Launches and Landings
- Spaceships and Rockets
- Communicating with Missions
- James Webb Space Telescope
- Hubble Space Telescope
- Why Go to Space
- Astronauts Home
- Commercial Space
- Destinations
Living in Space
- Explore Earth Science
- Earth, Our Planet
- Earth Science in Action
- Earth Multimedia
- Earth Science Researchers
- Pluto & Dwarf Planets
- Asteroids, Comets & Meteors
- The Kuiper Belt
- The Oort Cloud
- Skywatching
- The Search for Life in the Universe
- Black Holes
- The Big Bang
- Dark Energy & Dark Matter
- Earth Science
- Planetary Science
- Astrophysics & Space Science
- The Sun & Heliophysics
- Biological & Physical Sciences
- Lunar Science
- Citizen Science
- Astromaterials
- Aeronautics Research
- Human Space Travel Research
- Science in the Air
- NASA Aircraft
- Flight Innovation
- Supersonic Flight
- Air Traffic Solutions
- Green Aviation Tech
- Drones & You
- Technology Transfer & Spinoffs
- Space Travel Technology
- Technology Living in Space
- Manufacturing and Materials
- Science Instruments
- For Kids and Students
- For Educators
- For Colleges and Universities
- For Professionals
- Science for Everyone
- Requests for Exhibits, Artifacts, or Speakers
- STEM Engagement at NASA
- NASA's Impacts
- Centers and Facilities
- Directorates
- Organizations
- People of NASA
- Internships
- Our History
- Doing Business with NASA
- Get Involved
- Aeronáutica
- Ciencias Terrestres
- Sistema Solar
- All NASA News
- Video Series on NASA+
- Newsletters
- Social Media
- Media Resources
- Upcoming Launches & Landings
- Virtual Events
- Sounds and Ringtones
- Interactives
- STEM Multimedia
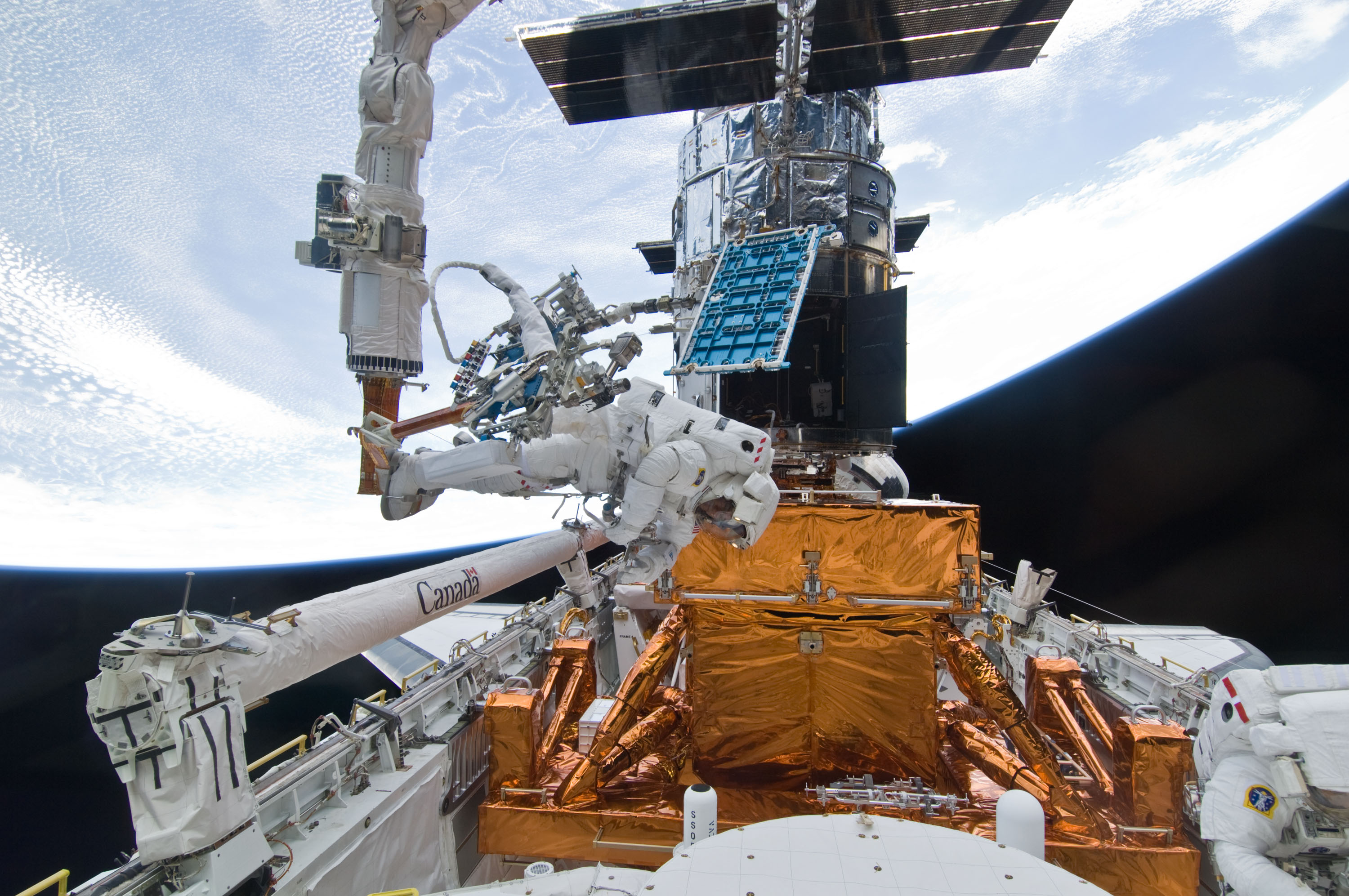
Hubble Celebrates the 15th Anniversary of Servicing Mission 4
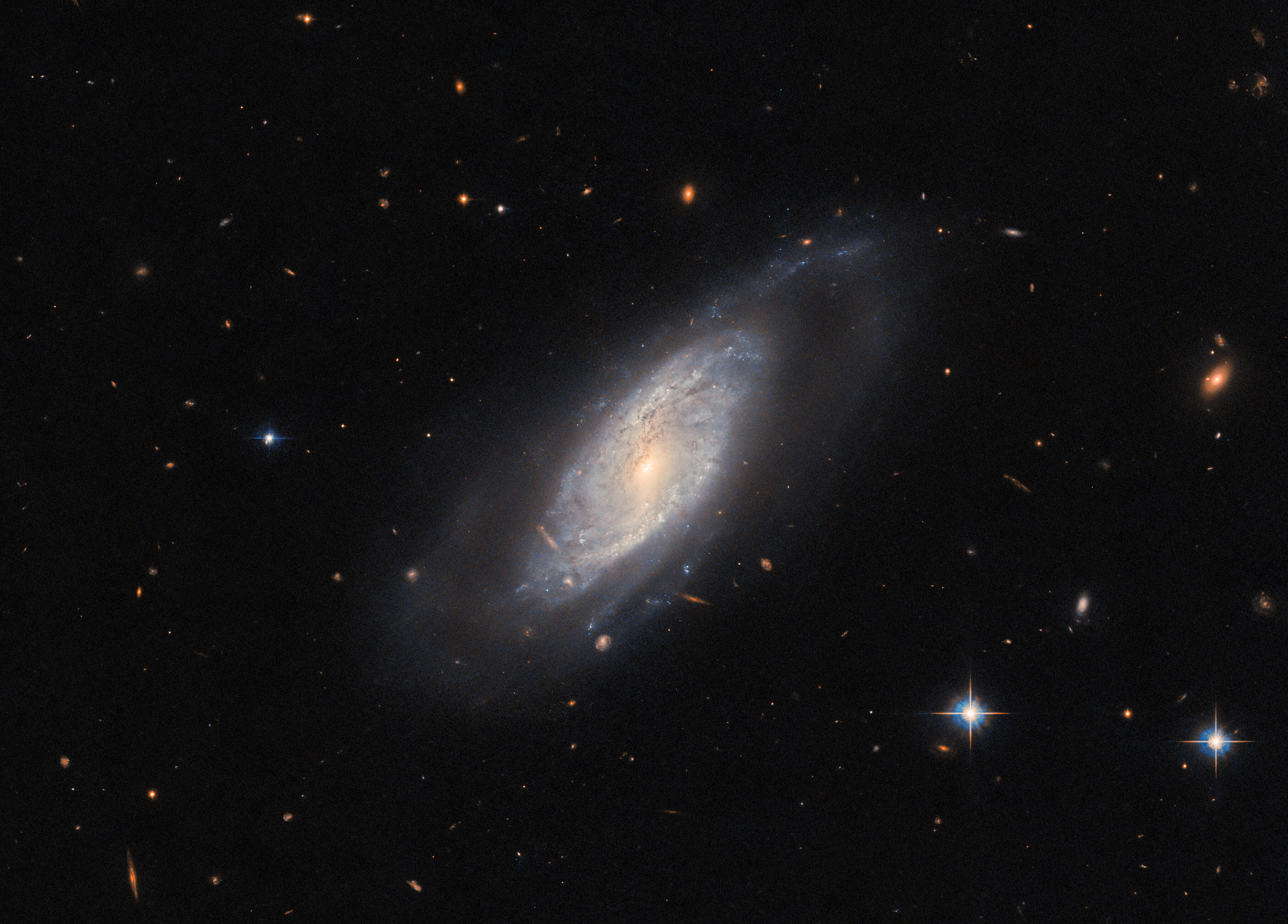
Hubble Glimpses a Star-Forming Factory
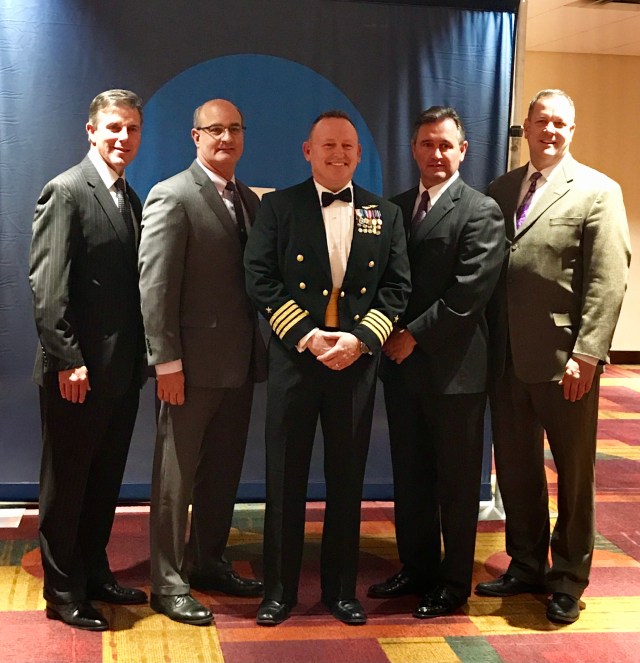
NASA Mission Strengthens 40-Year Friendship
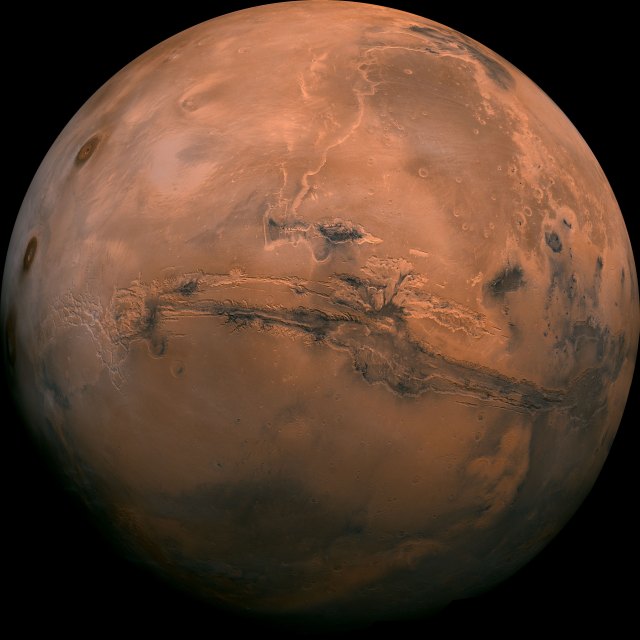
NASA Selects Commercial Service Studies to Enable Mars Robotic Science
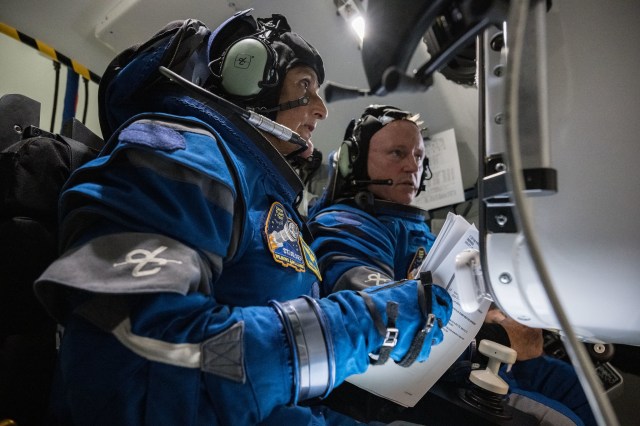
NASA’s Commercial Partners Deliver Cargo, Crew for Station Science
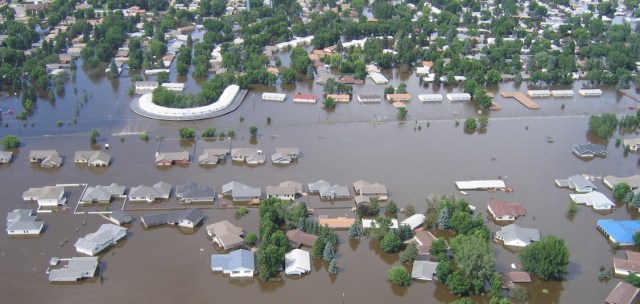
International SWOT Mission Can Improve Flood Prediction
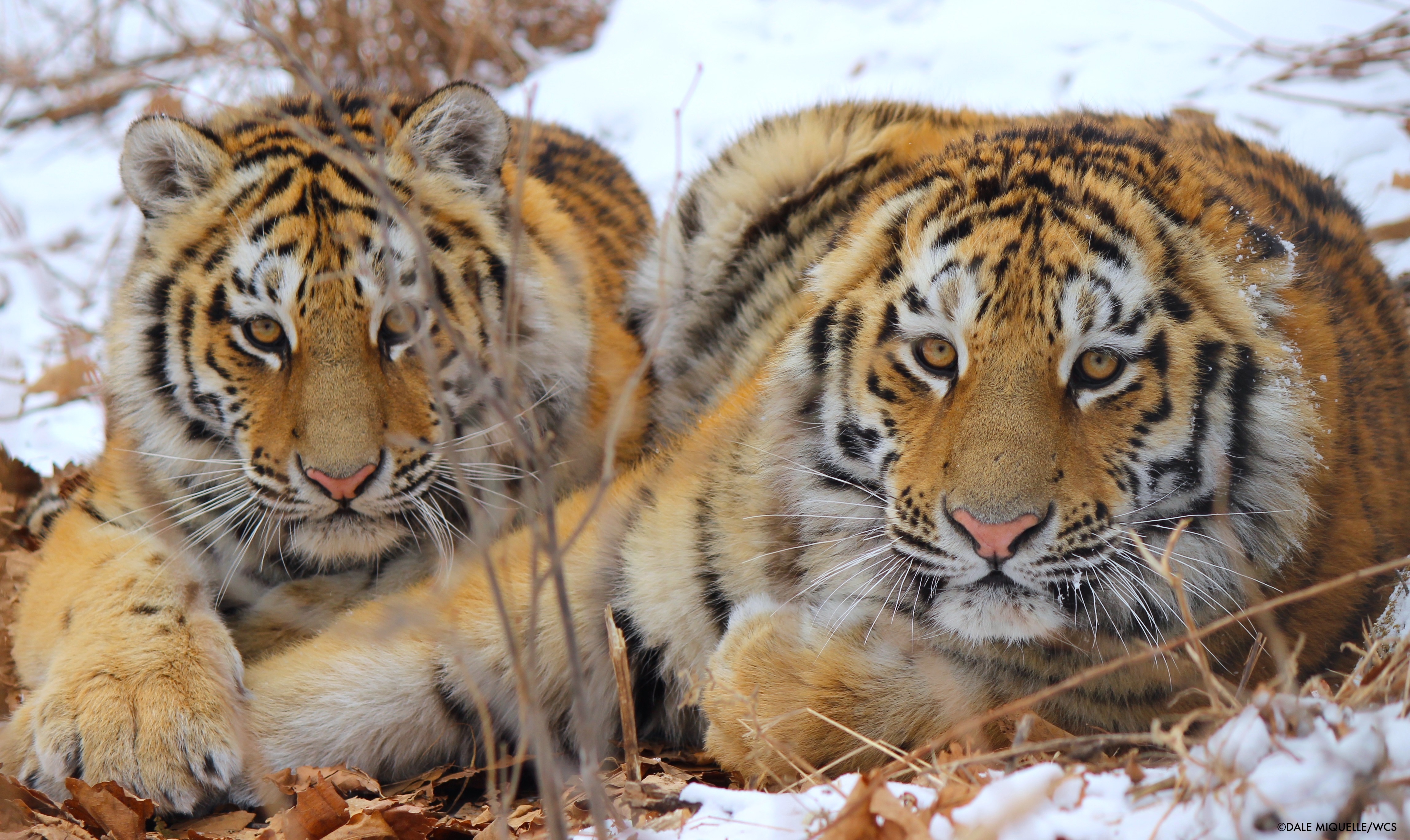
NASA Is Helping Protect Tigers, Jaguars, and Elephants. Here’s How.
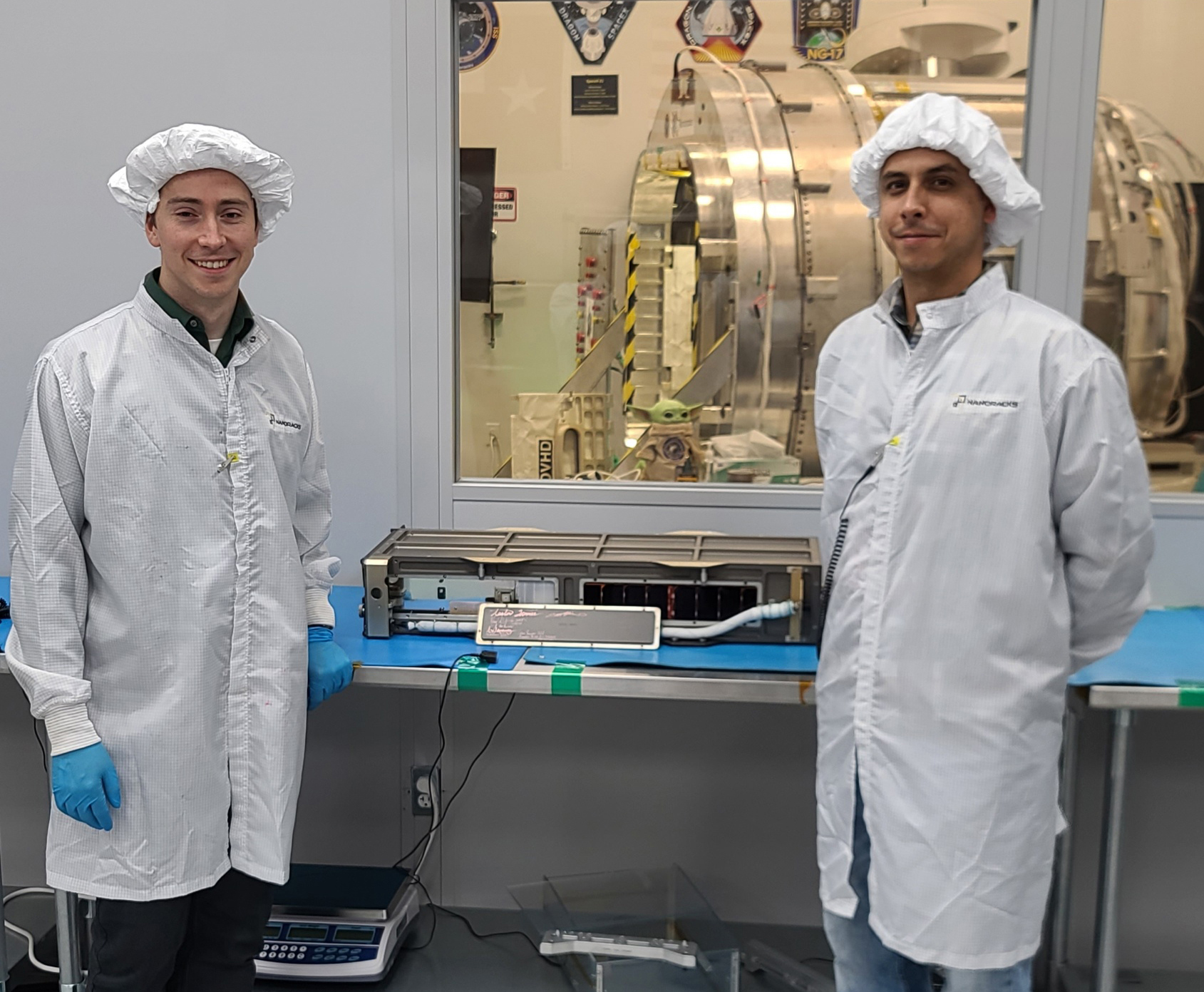
Two Small NASA Satellites Will Measure Soil Moisture, Volcanic Gases
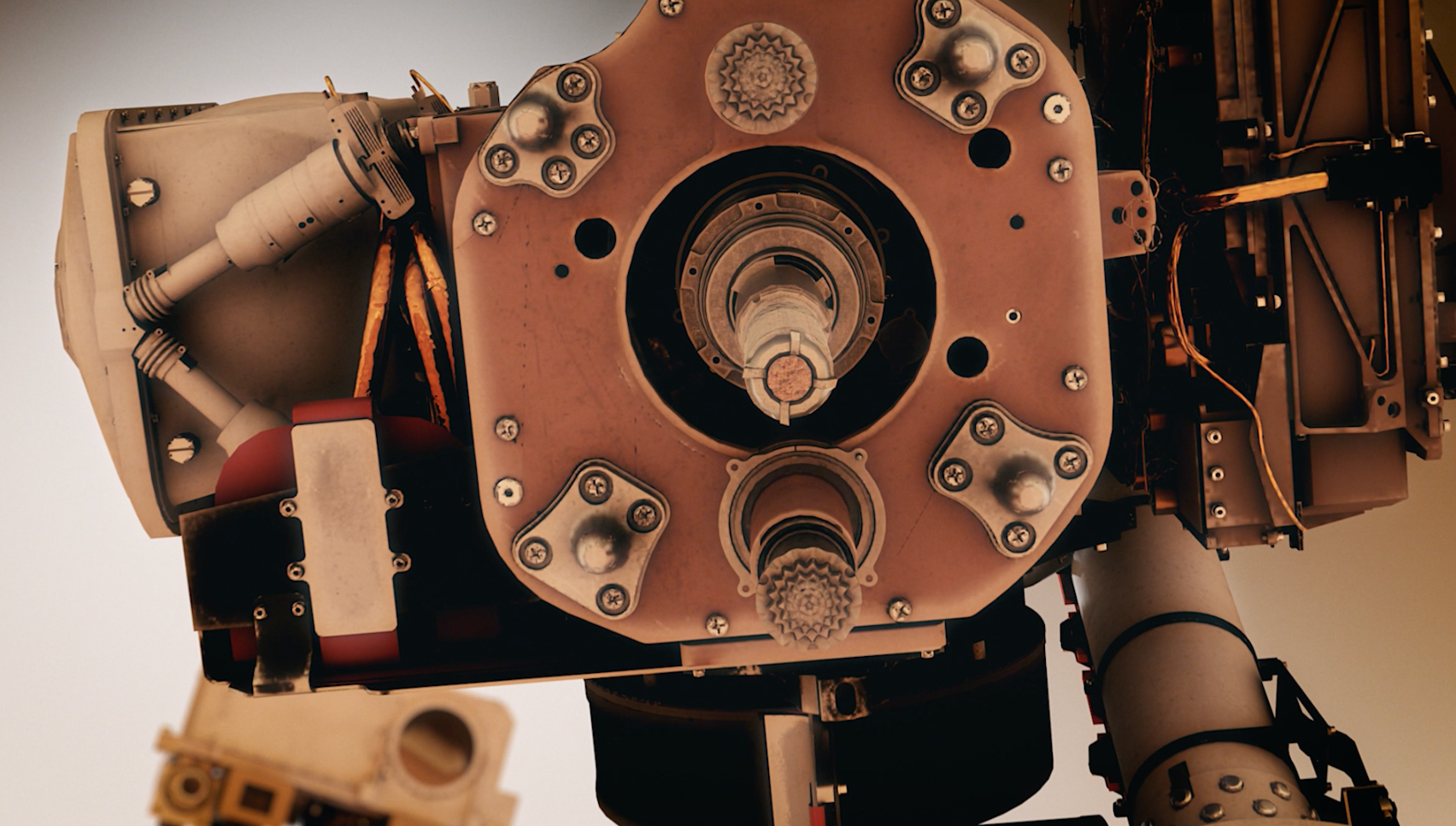
C.26 Rapid Mission Design Studies for Mars Sample Return Correction and Other Documents Posted
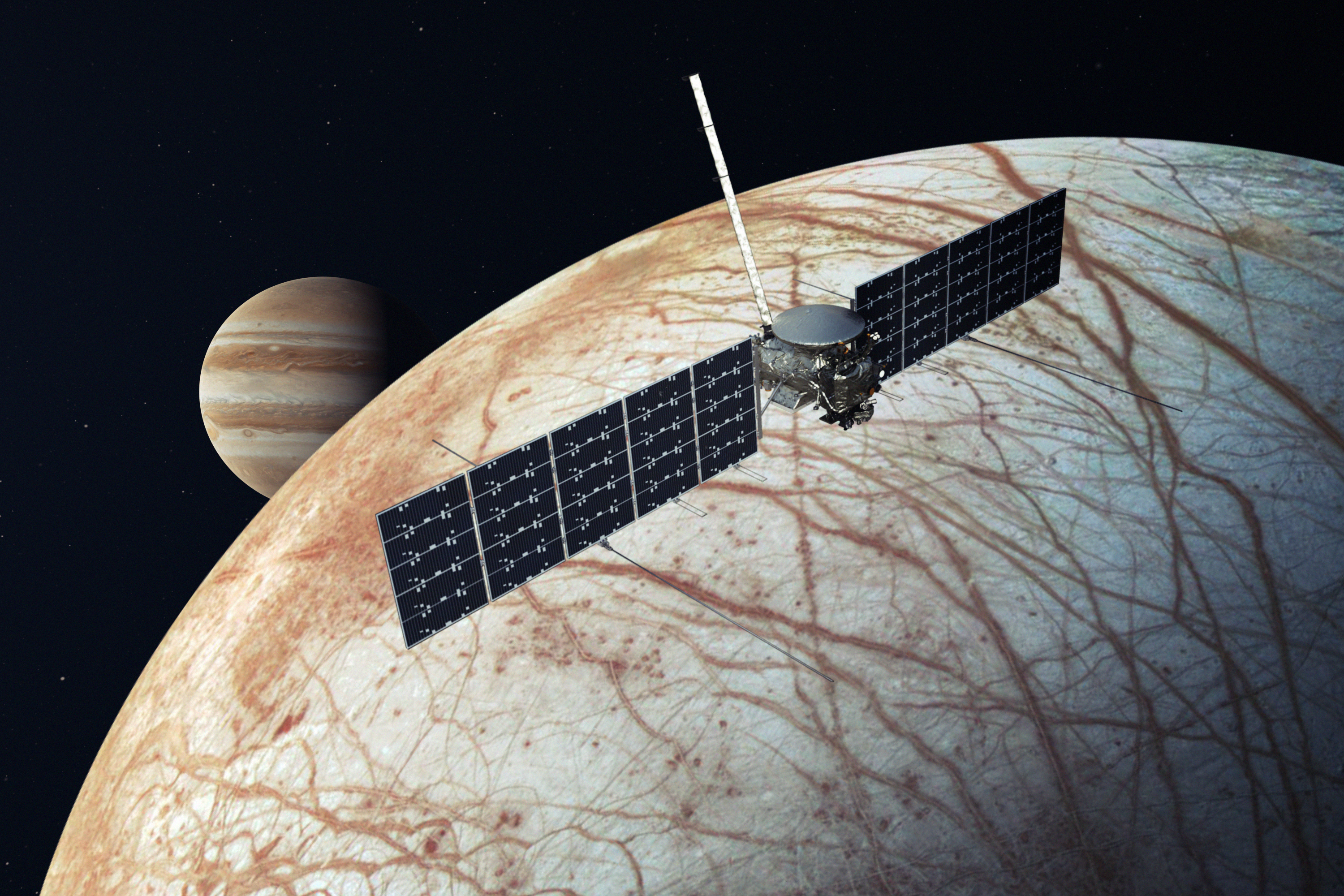
NASA Selects Students for Europa Clipper Intern Program
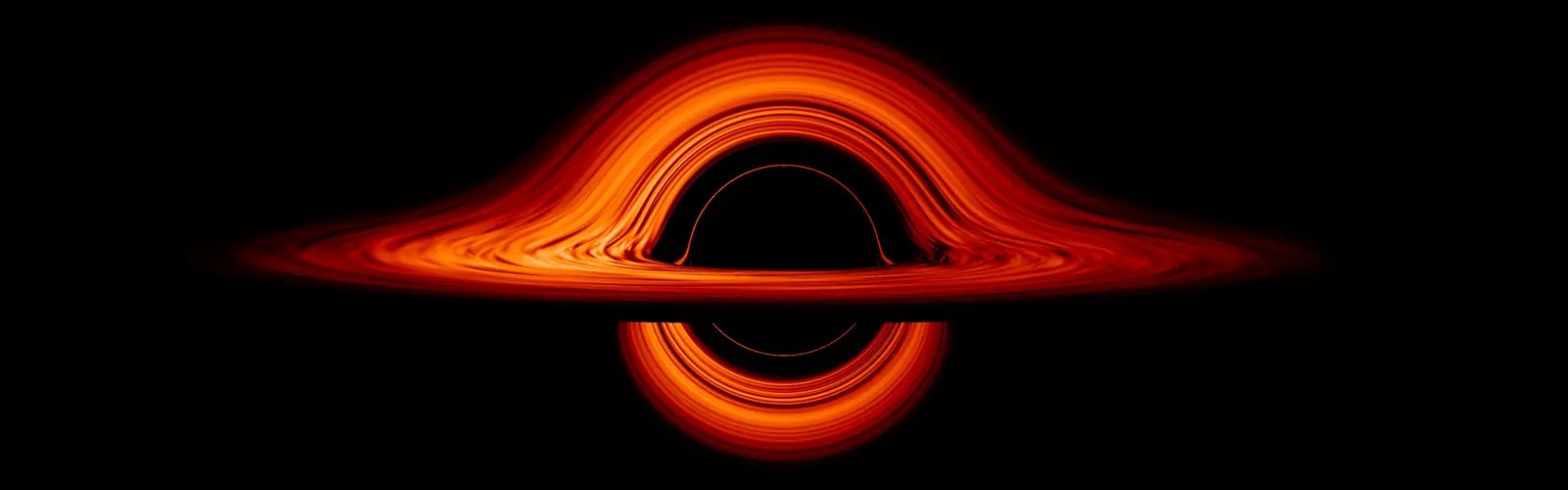
The Big Event, 2024
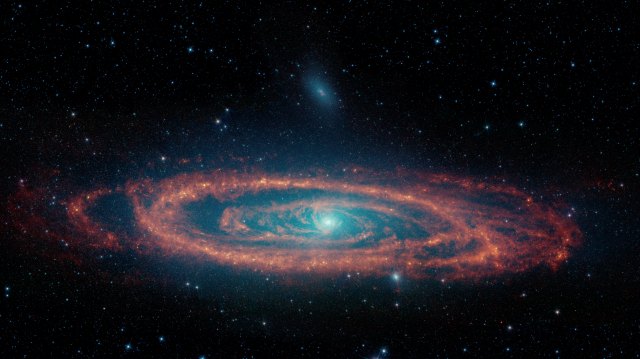
NASA Images Help Explain Eating Habits of Massive Black Hole
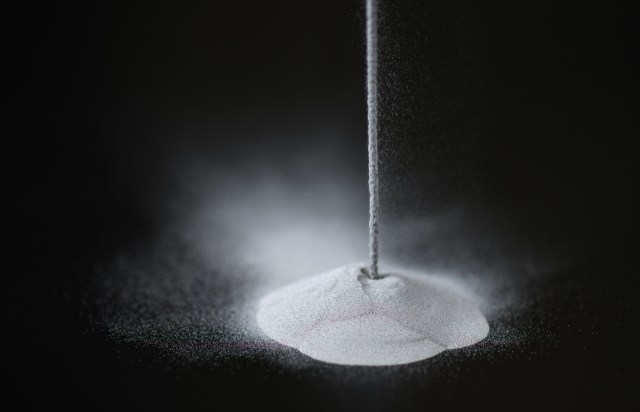
NASA Licenses 3D-Printable Superalloy to Benefit US Economy
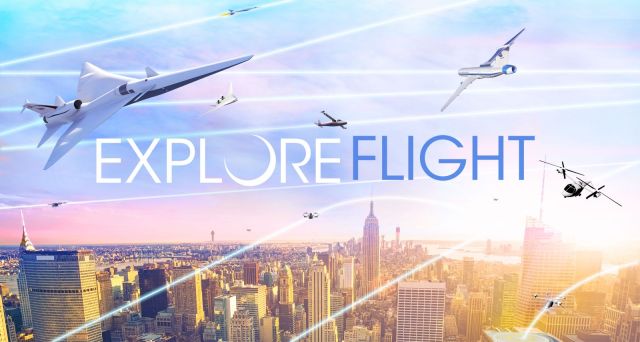
ARMD Solicitations

NASA’s Commitment to Safety Starts with its Culture
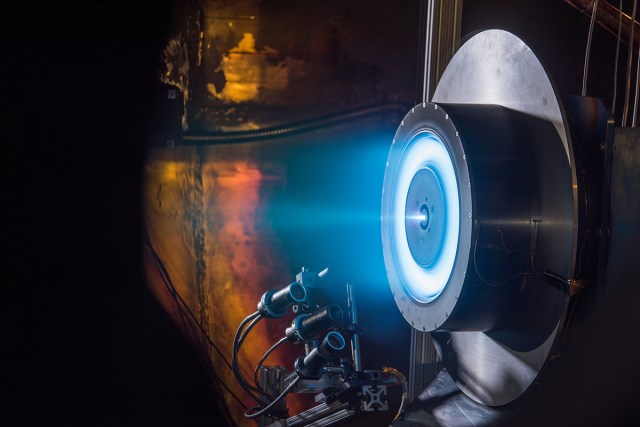
Tech Today: NASA’s Ion Thruster Knowhow Keeps Satellites Flying
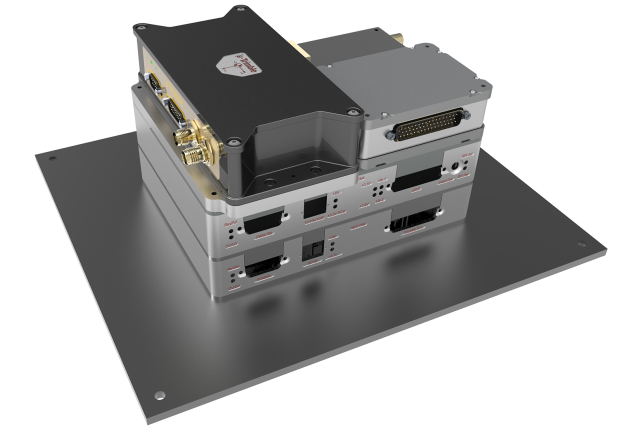
Big Science Drives Wallops’ Upgrades for NASA Suborbital Missions
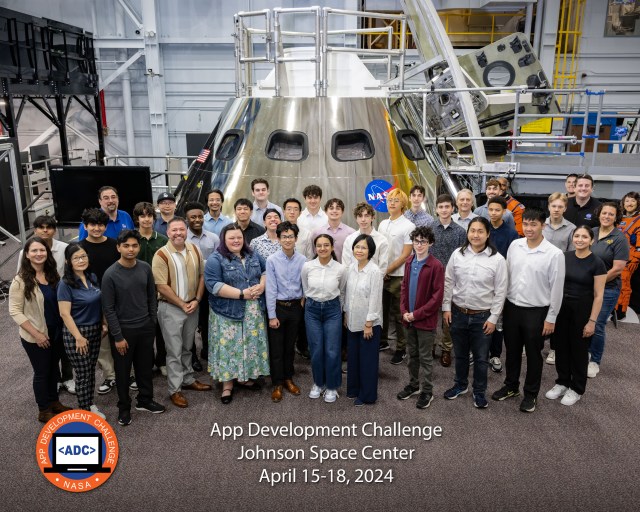
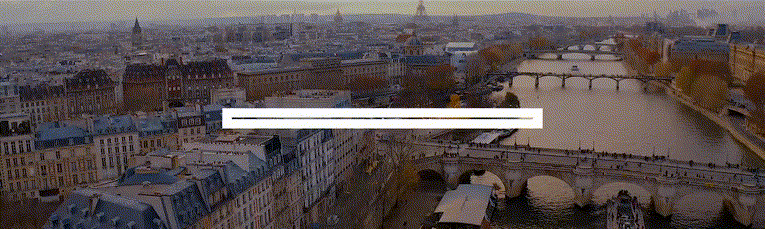
NASA Challenge Gives Artemis Generation Coders a Chance to Shine
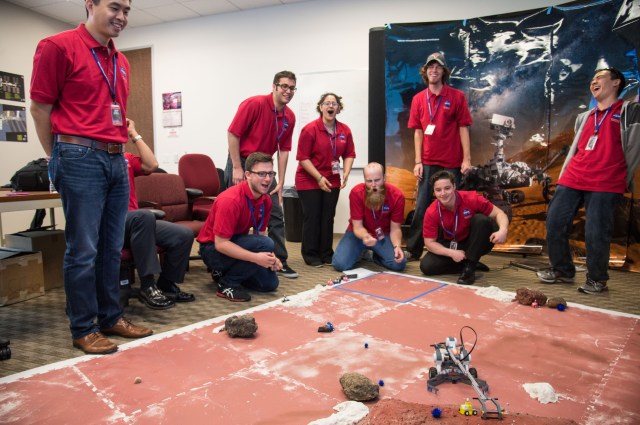
NASA Community College Aerospace Scholars
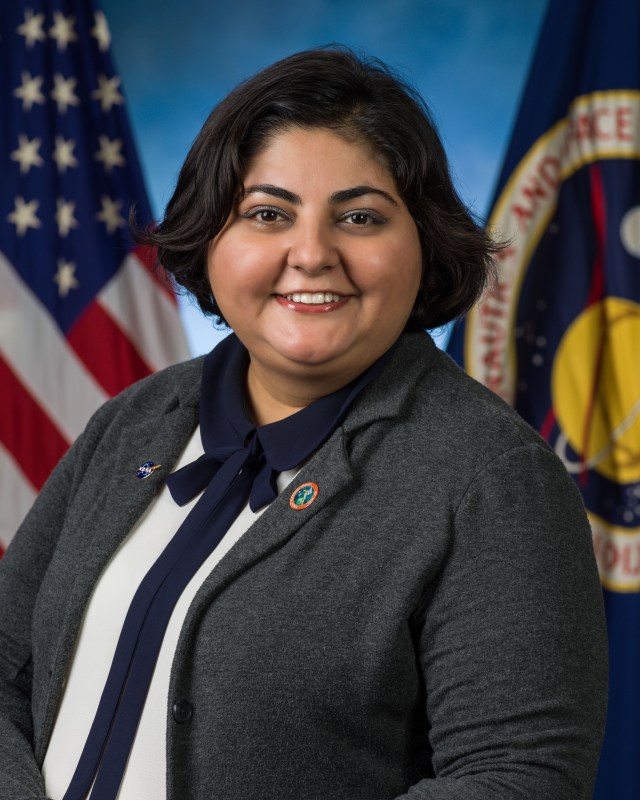
Johnson Celebrates AA and NHPI Heritage Month: Kimia Seyedmadani
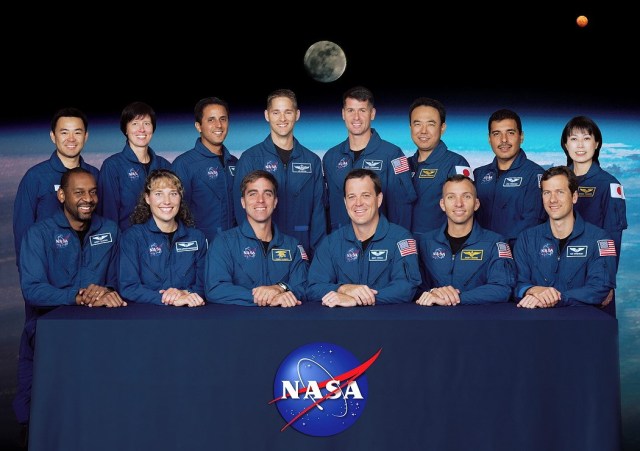
20 Years Ago: NASA Selects its 19th Group of Astronauts
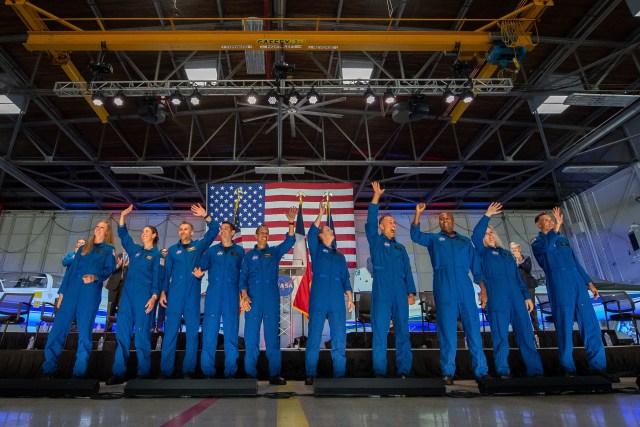
Diez maneras en que los estudiantes pueden prepararse para ser astronautas
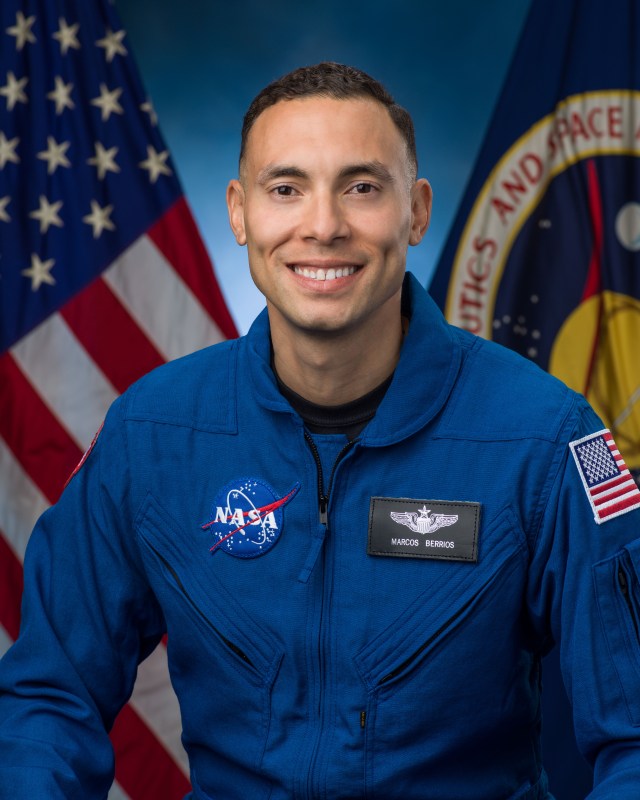
Astronauta de la NASA Marcos Berríos
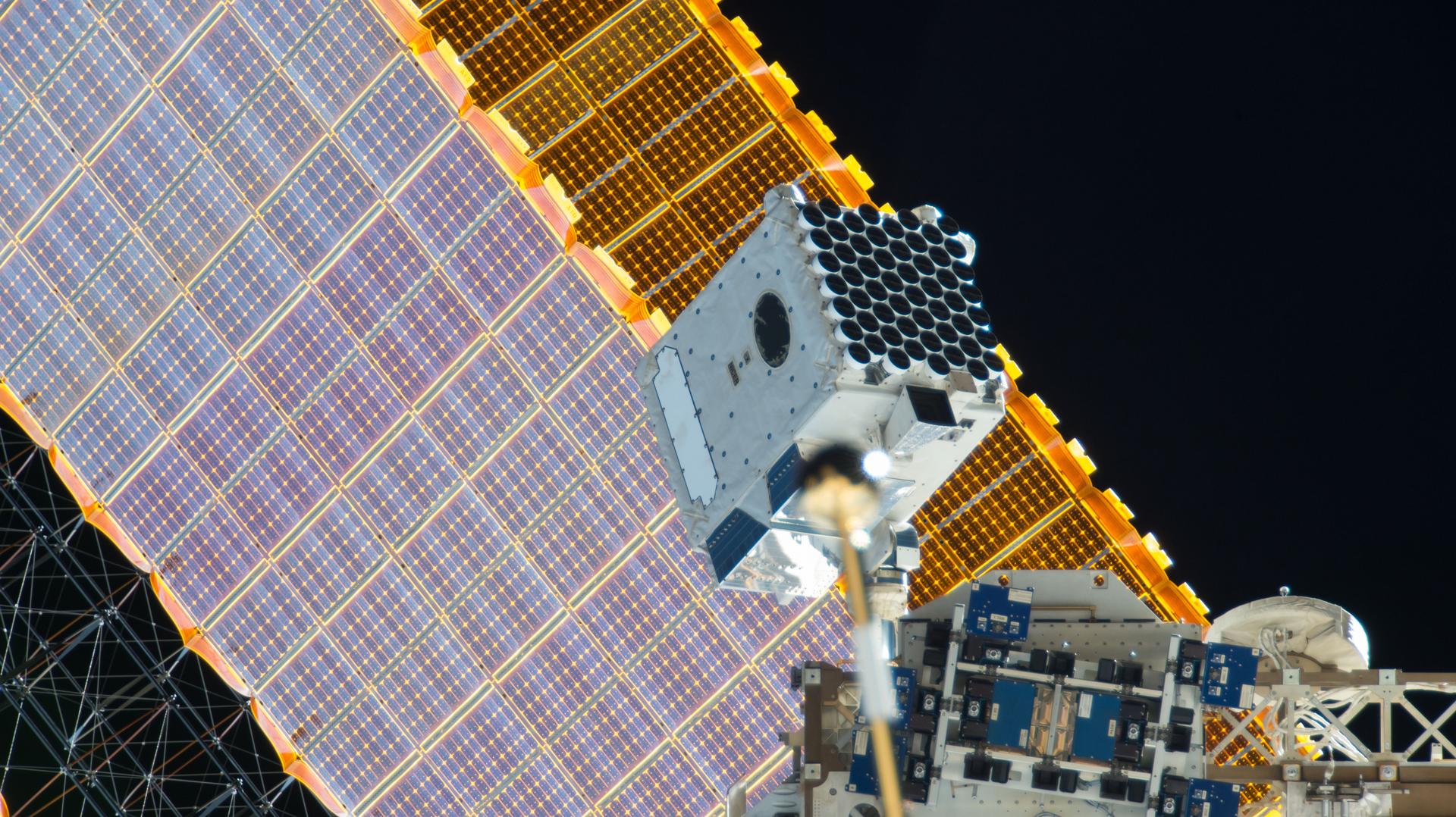
Resultados científicos revolucionarios en la estación espacial de 2023
Experiments to unlock how human bodies react to long space journeys.
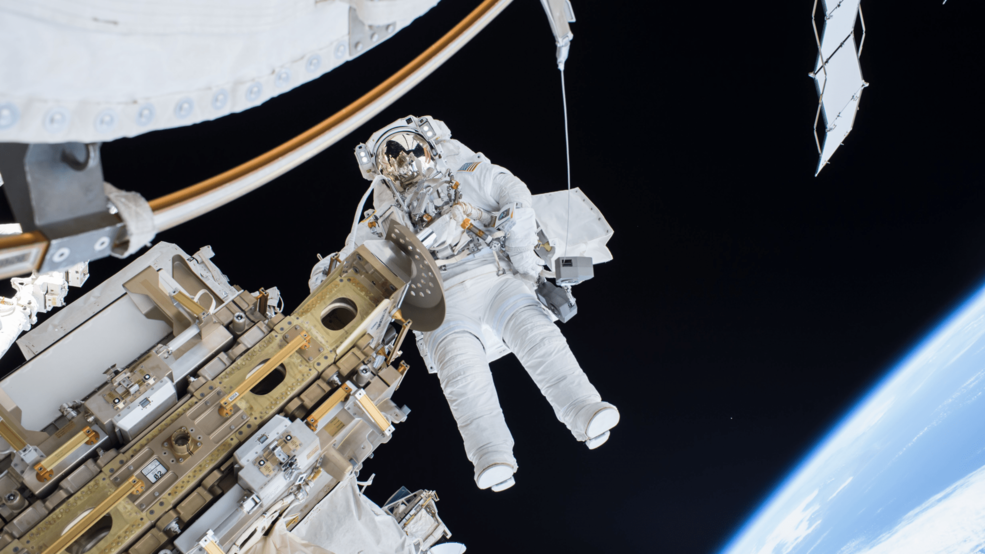
Nathan Cranford
Jennifer Turner
Bone and joint health, brain and behavior, cardiovascular, sensorimotor.
Through Artemis , NASA astronauts are returning to the Moon in preparation for one day going to Mars. To better prepare astronauts for these long journeys, scientists need to know: How do extended durations in space change the human body?
Astronauts flying to the International Space Station can now volunteer for a suite of experiments that aim to help scientists learn more. Together, these experiments are called the Complement of Integrated Protocols for Human Exploration Research, or CIPHER.
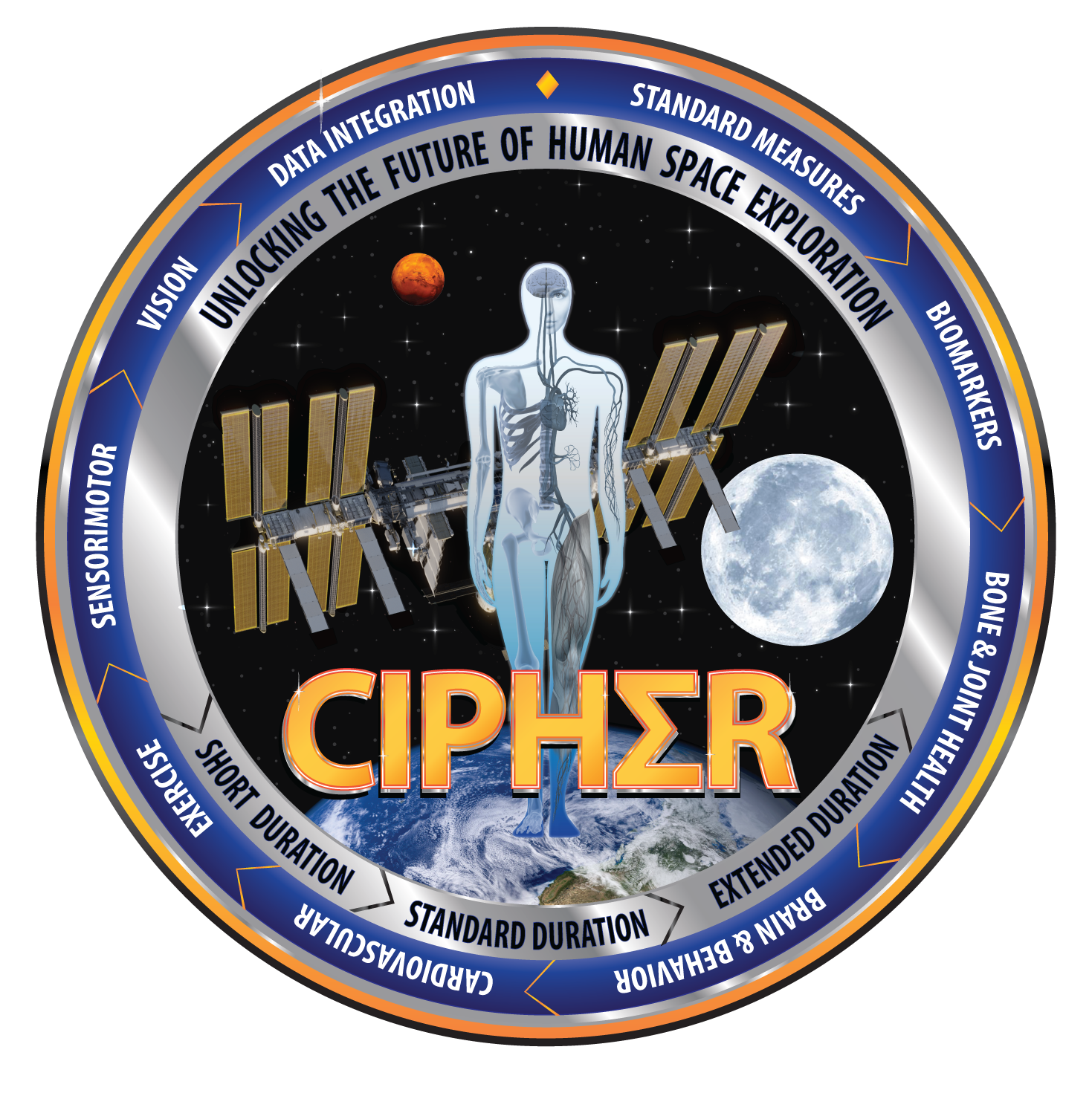
Through CIPHER, astronauts participate in an integrated set of 14 studies sponsored by NASA and international partner agencies. To get meaningful results, CIPHER scientists will study up to 30 astronauts, evenly divided over three mission-length categories:
- Short (less than 3.5 months in space)
- Standard (between 3.5 and eight months in space)
- Extended (more than eight months in space)
These research studies will monitor the health of astronauts before, during, and after their missions, and together address the following themes.
Studies show that astronauts lose bone density and muscle quality faster in space than on Earth. Calcium lost from bone ends up in their blood and urine. This loss of bone density may affect how skeletal systems support muscles and organs in space and soon after landing back on Earth.
Astronauts will:
- Undergo scans to measure bone density, skeletal health, and muscle quality surrounding bones and joints; and
- Collect blood and urine periodically before, during, and after their missions.
Scientists will:
- Examine whether the rate of bone and muscle loss stays constant, slows down, or even stops beyond six-month missions; and
- Determine what sort of health risks, if any, these pose.
Fluids in the brain shift due to low gravity in space, and long-duration spaceflight may slightly alter brain structure. These changes could affect how the brain processes spatial information and, in turn, affect crew performance.
- Complete cognitive tests,
- Perform spatial cognition tasks using virtual reality,
- Use a computer to measure proficiency in controlling a robotic arm, and
- Undergo MRI scans while doing cognitive tests.
- Examine how brain activity before missions differs from brain activity after missions,
- Pinpoint patterns dependent on mission duration, and
- Assess how changes in performance of cognitive, spatial cognition, and operational tasks relate to changes in brain structure and function.
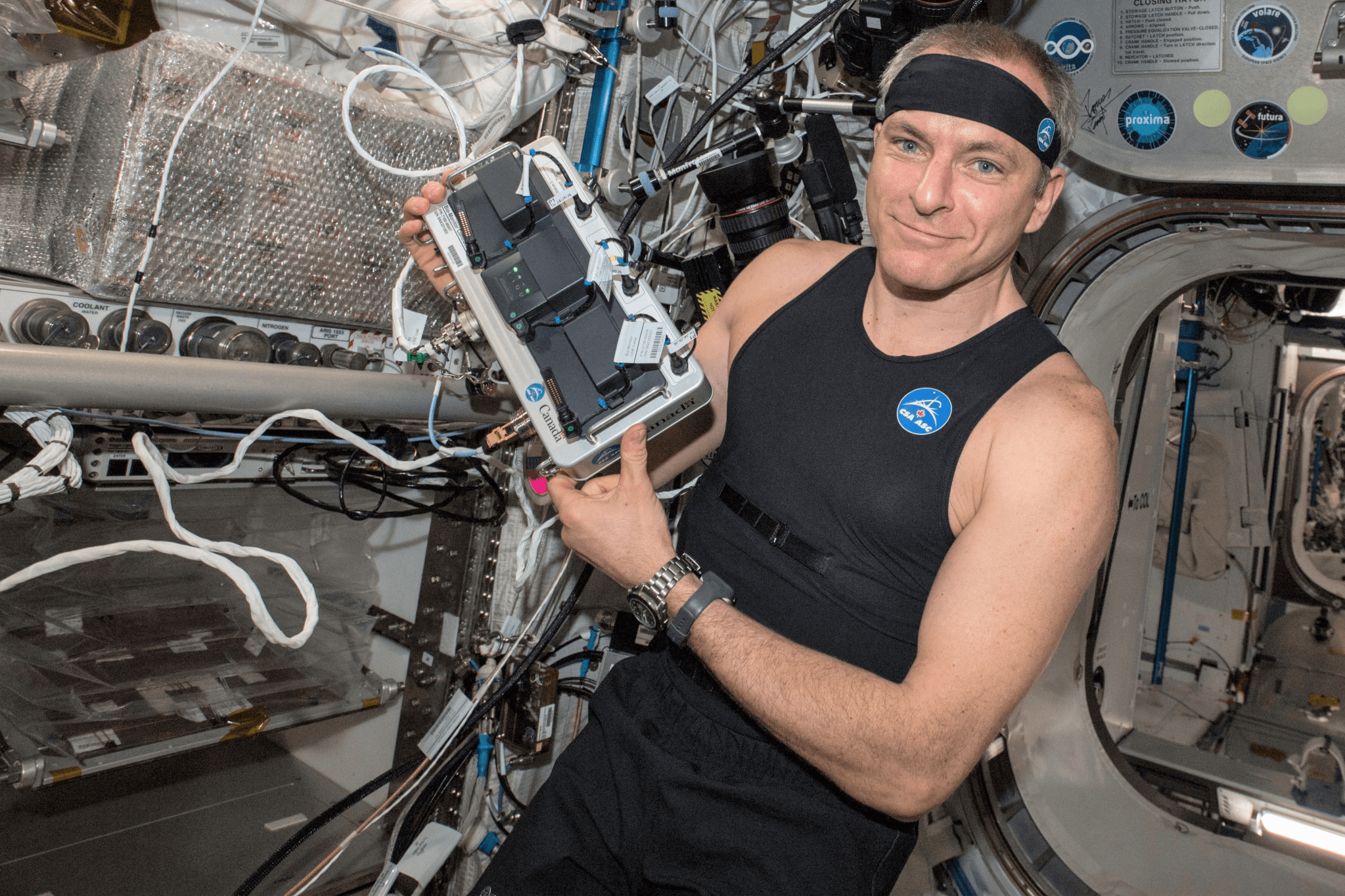
Long-duration spaceflight may lead to stiffer arteries and increase the risk of heart disease. Scientists want to know how these changes play out during and after extended missions.
- Complete CT, MRI, and ultrasound imaging of the heart, surrounding organs and muscles, and blood vessels,
- Wear a shirt that measures heart rate and respiration across two days, and
- Take periodic blood pressure measurements.
- Tease out patterns within and across mission durations to provide clear, objective indicators of cardiovascular health, and
- Examine whether the low-gravity affects organs, muscles, and vessels near the heart.
Exercise is a well-known strategy to counter bone and muscle loss in space. Researchers want to understand whether exercise is an effective strategy for maintaining astronaut health in long-duration spaceflight.
- Test muscle strength and endurance throughout their missions using the station’s exercise equipment,
- Track their nutrition and sleep habits, and
- Navigate through an obstacle course after they land back on Earth, while at times wearing spacesuits connected to NASA’s Active Response Gravity Offload System, or ARGOS, to simulate Martian gravity.
- Evaluate crews’ abilities to carry out specific exercises over time, and
- Evaluate how soon and how well crews can perform critical tasks in different gravities.
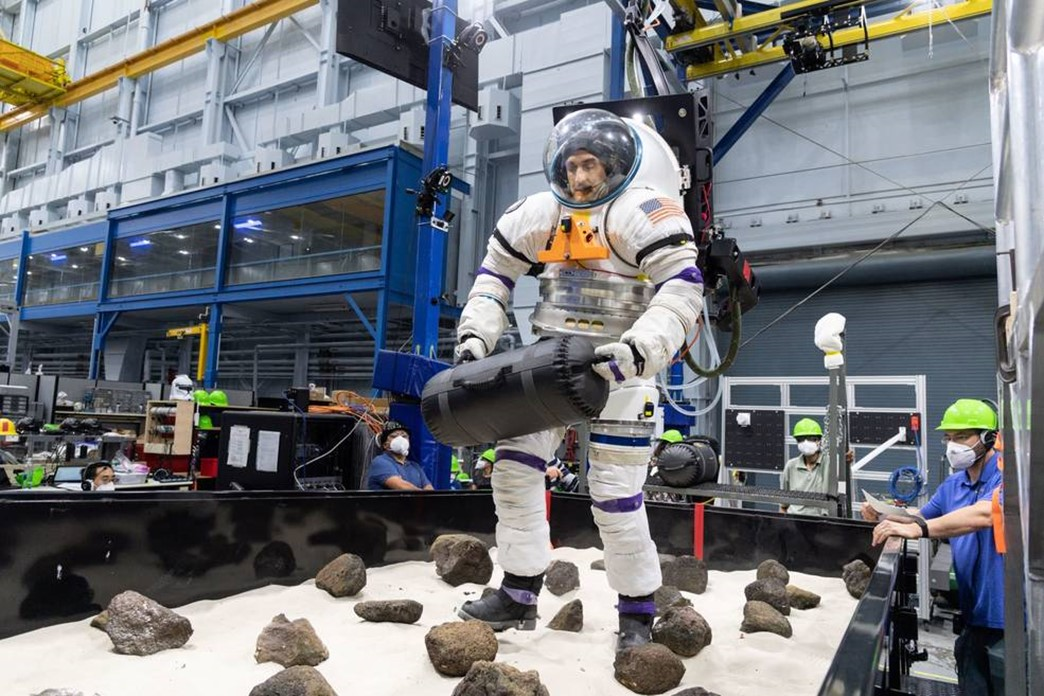
Many astronauts experience dizziness and disorientation when they arrive at the station and when they return to Earth. However, on future missions to Mars, ground support won’t be readily available to assist astronauts when they land on the Red Planet’s surface. Understanding factors that may influence how long these symptoms last will be important to mission success.
- Record their eye, head, and body movements using specialized techniques, and
- Fill out surveys on how they perceive motion.
- See whether mission duration influences how long it takes to adapt to a different gravity level, and
- Investigate the reasons behind changes in balance and the ability to adapt to different gravities.
Because low-gravity conditions shift the body’s fluids toward the head, spaceflight can alter the structure and function of the eyes and the brain. This, in turn, may influence how astronauts process visual information on long missions, which could affect their performance.
- Participate in MRI and eye imaging scans,
- Perform vision tests, and
- Assess eye pressure and how the retina responds to light.
- Evaluate eye changes for each mission duration, and
- See how the structure of astronauts’ brains change after their missions, and how such changes affect vision.
Samples of astronaut’s blood and urine can provide insights into crew health. For instance, stress hormones reveal how the immune system reacts to spaceflight. Scientists want to know whether any changes to the human body induced by long-duration space travel come with indicators that can also be detected in blood and urine.
- Provide blood and urine samples before, during, and after spaceflight, and
- Complete questionnaires regarding health and exercise habits.
- Examine blood and urine for potential indicators of changes to cartilage health, inflammation and immune function, kidney health, brain structure and function, spatial cognition, performance of operational tasks, risk of cardiovascular disease, and more; and
- Investigate the interplay between mission length, DNA damage responses, and post-mission recovery of telomeres – the caps at the ends of chromosomes that shorten as we age but may lengthen in space before rebalancing themselves back on Earth.
CIPHER also includes a long-running study called Spaceflight Standard Measures , which collects a core set of information on as many crew members as possible. This core set includes metrics about the crew member’s sleep, cognition, biomarkers, immune function, microbiome, and more.
In addition to answering research questions central to each study, CIPHER takes an integrated approach – data across the CIPHER investigations will be evaluated to identify patterns and gain a deeper understanding of how the human body reacts to long durations in space. For instance: Do changes to the various systems of the body plateau across the board after specific amounts of time spent in space? Do changes in one system herald changes in another?
“CIPHER is an all-encompassing, total-body approach to learning how humans adapt to spaceflight,” says Oubre. “Insights gained through CIPHER may well be key to enabling humans to remain healthy while exploring the Moon, Mars, and beyond.”
NASA is leading a return to the Moon for long-term exploration. Through the Artemis missions, NASA will land the first woman and first person of color on the Moon, using innovative technologies to explore more of the lunar surface than ever before. Lessons learned on and around the Moon will prepare NASA for the next giant leap: sending astronauts to Mars.
Learn more about Artemis here.
NASA’s Human Research Program , or HRP, pursues the best methods and technologies to support safe, productive human space travel. Through science conducted in laboratories, ground-based analogs, and the International Space Station, HRP scrutinizes how spaceflight affects human bodies and behaviors. Such research drives HRP’s quest to innovate ways that keep astronauts healthy and mission-ready as space travel expands to the Moon, Mars, and beyond.
Learn more about the Human Research Program here .
Related Terms
- CIPHER (Complement of Integrated Protocols for Human Exploration Research)
Human Research Program
Explore More
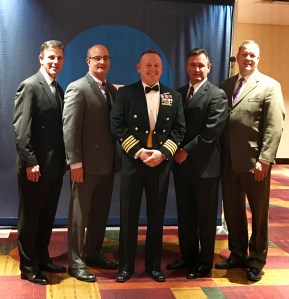
Discover More Topics From NASA
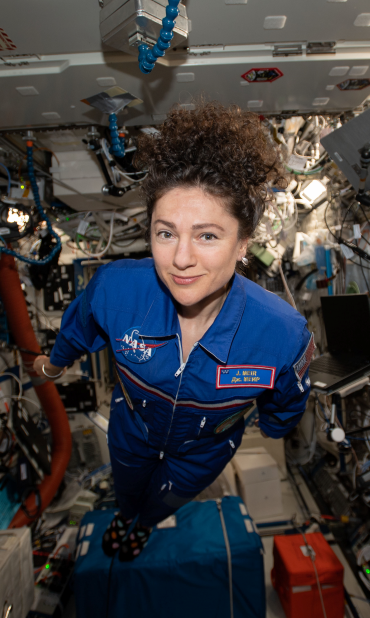
Space Station Research and Technology
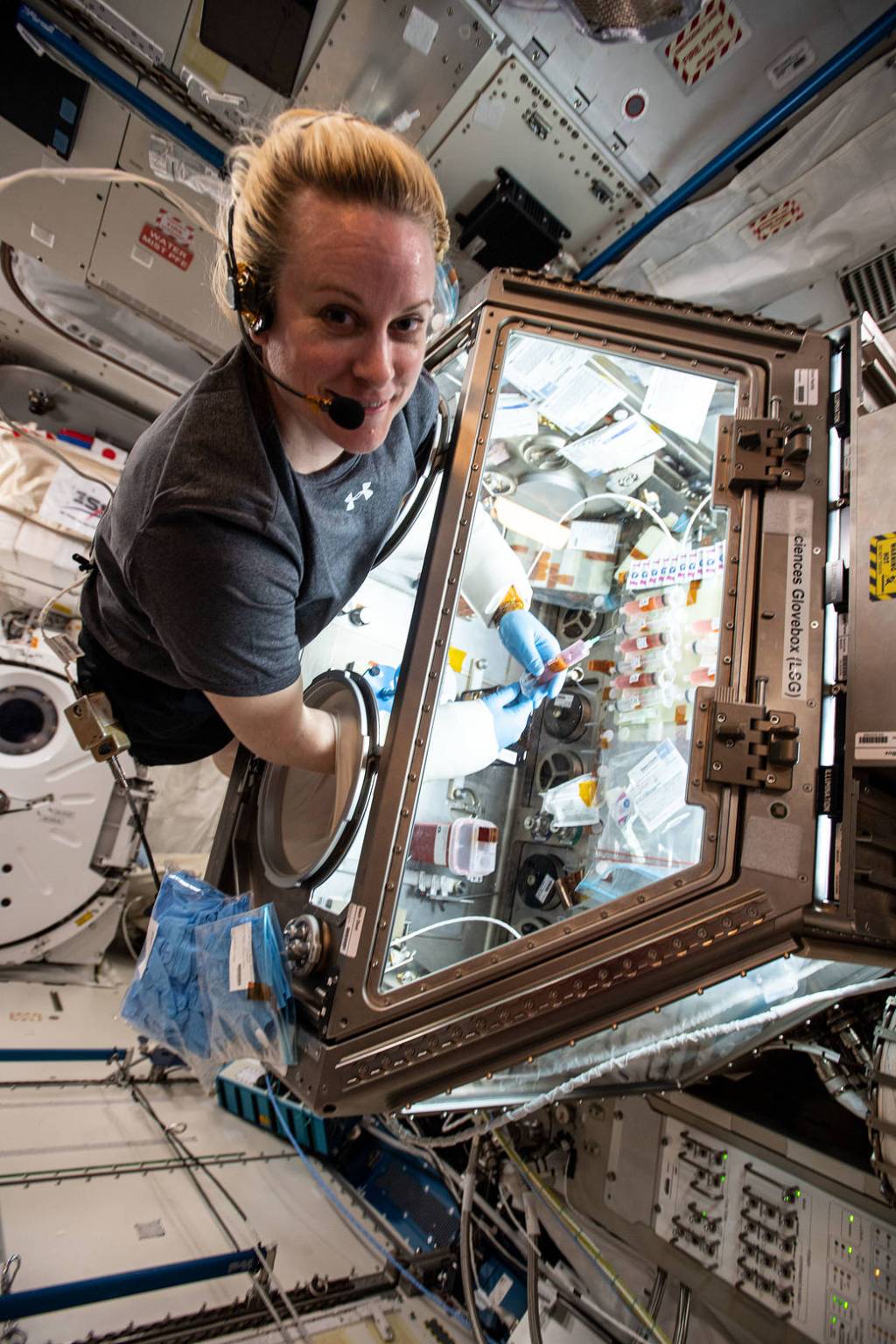
We have completed maintenance on Astronomy.com and action may be required on your account. Learn More
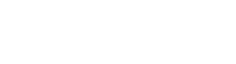
- Login/Register
- Solar System
- Exotic Objects
- Upcoming Events
- Deep-Sky Objects
- Observing Basics
- Telescopes and Equipment
- Astrophotography
- Space Exploration
- Human Spaceflight
- Robotic Spaceflight
- The Magazine
How vast is the universe? Unimaginably enormous
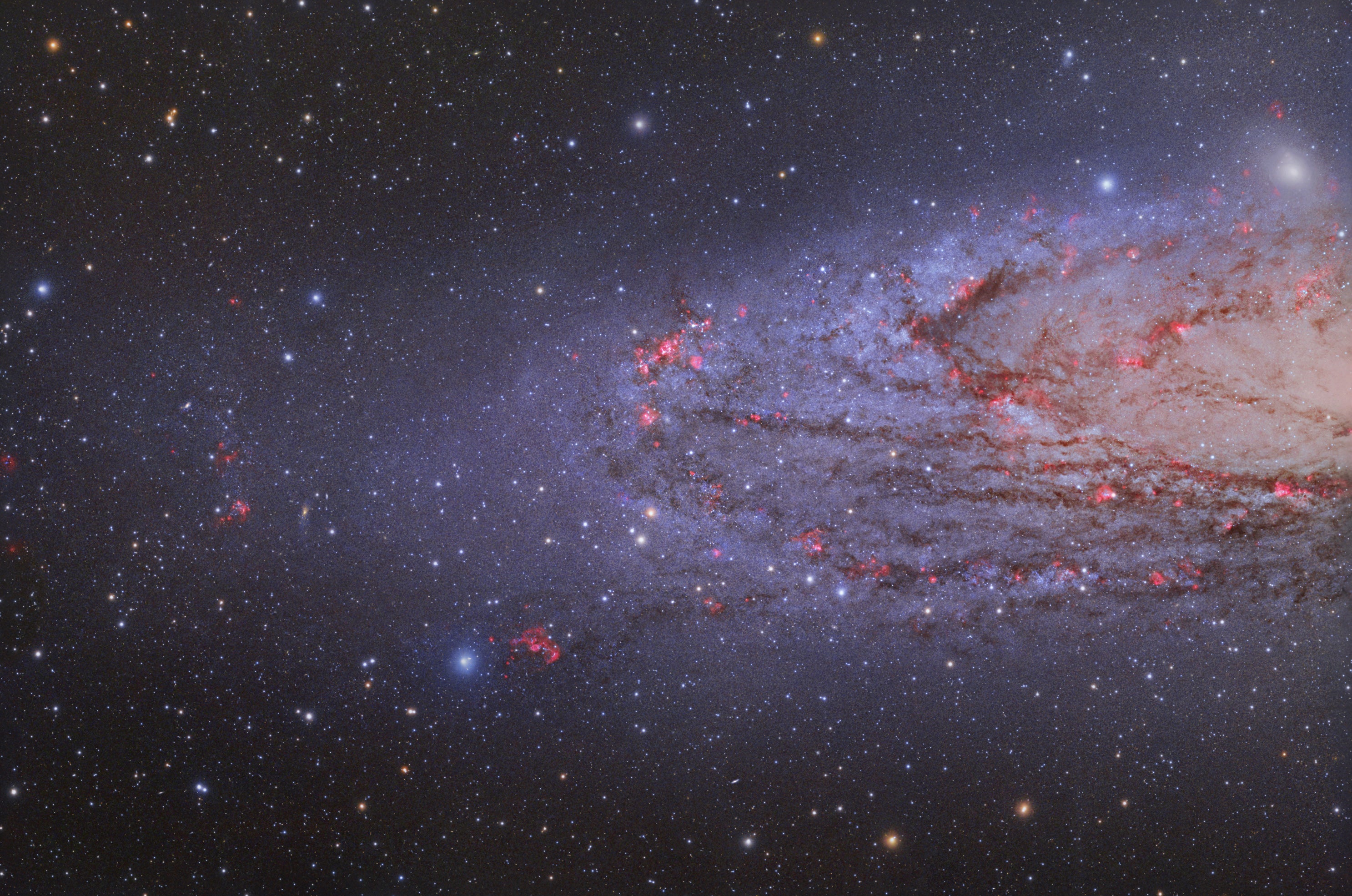
Many people, even astronomy enthusiasts, routinely wonder about the frequent reports of UFOs, or UAPs (unidentified anomalous phenomena), frequently spotted in the sky and sending people into fits over alien visitation. But the people who are sincerely worried about that have never taken the first week of an academic course on what astronomers call the cosmic distance scale.
In the words of the popular writer Douglas Adams, author of A Hitchhiker’s Guide to the Galaxy , “Space is big. You just won’t believe how vastly, hugely, mind-bogglingly big it is. I mean, you may think it’s a long way down the road to the chemist’s, but that’s just peanuts to space.”
And Adams is exactly right. Let’s begin by considering local space. Assume that the distance between Earth and the Sun, known as 1 astronomical unit, is 1 centimeter instead of its actual 93 million miles. In all of human history, mind you, we have physically traveled an imperceptibly small fraction, then, of that 1 centimeter.
Comparing cosmic distance scale
You can think of a scale model solar system in your room, your neighborhood, and your town. On that scale, Jupiter would be 5 cm from the Sun. Keep moving off, say, to the right, and imagining the relative distances. Saturn would be 10 cm from the Sun, Uranus 19 cm from the Sun, and Neptune 30 cm from the Sun. Little old Pluto would typically be about 40 cm from the Sun.
On this scale, however, the physical diameter of the solar system is far larger than that. The outer edge of the Oort Cloud, the enormous shell of perhaps a trillion comets, would lie 10 football fields away. And we have, thus far, traveled a tiny, almost invisible fraction of that original 1 cm.
And that’s just our solar system. The nearest star, Proxima Centauri, is four times farther away than the outer shell of the Oort Cloud. The enormous gap between even the nearest stars would be staggeringly large. The actual distance to Proxima Cen is 4.2 light-years, meaning that light — the fastest thing there is — takes 4.2 years to travel from that star to our eyes.
RELATED: How astronomers measure an infinite universe
Beyond the Milky Way
We can take quantum leaps upward in universal scale, too. The Milky Way Galaxy is one of at least 100 billion galaxies in the cosmos. The diameter of the Milky Way’s bright disk, which we are in, is at least 100,000 light-years. (We are a little more than 26,000 light-years out from the galaxy’s center.) The most distant photons from our own galaxy have been traveling to us for one-third of the length of human history.
And a little like nesting dolls, we can follow cosmic structures out to larger and larger scales. Our Local Group of galaxies , as Edwin Hubble termed it back in the 1930s, contains at least 55 galaxies in a sphere spanning about 10 million light-years. Local Group members include our nearby friends the Andromeda Galaxy and the Triangulum Galaxy .
Countless small groups of galaxies are even farther away, and if we travel some 55 million light-years, we get to the center of the largest cluster of galaxies in our cosmic space, the Virgo Cluster . This group includes some 1,300 galaxies of all types. But larger and more massive groups of galaxies lie far more distantly from us. Nearly countless numbers of them.
When we get up to the largest cosmic structures known, we can talk about superclusters and walls of galaxy clusters. Examples include Laniakea, which we belong to, a structure that stretches some 500 million light-years across. And there are dozens of examples of other large structures.
The universe could, indeed, be infinite
How large is the universe? Put simply, we don’t know. The best current estimate is that the universe’s diameter is at least 93 billion light-years. And if cosmic inflation is correct (a long story, but a likelihood), then the visible universe that we see may not be the whole thing. As much as it sounds like science fiction, the universe could actually be infinite in size. And of course whatever its size, we know that the universe is expanding over time, and that this expansion is accelerating over time as well.
Thankfully, we can see the distant universe as it was long ago because photons are massless, and they travel at 186,000 miles per second, the fastest speed there is. The folks who are perplexed or anguished by reports of UFOs not only don’t appreciate the unbelievably vast cosmic distance scale. They also don’t understand that only massless objects, photons, can travel at such high velocities. Things with mass require a tremendous amount of energy to convert into high-speed motion. Don’t believe me? Try eating a tuna sandwich and running around the circumference of Earth a few times before taking up a rest. You’ll get it then.
So the universe may be teeming with life (that’s a topic for another time), but it is so distant that we will not encounter it staring us in the face, accompanying us to lunch in Central Park. That’s the stark reality that trained astronomers know.
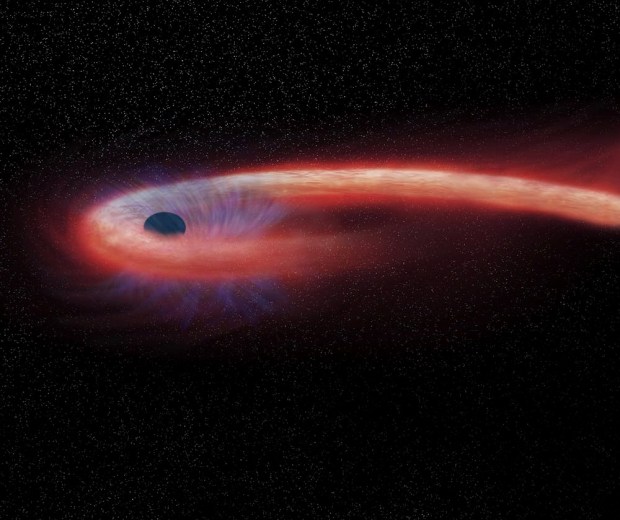
IceCube researchers detect a rare type of particle sent from powerful astronomical objects
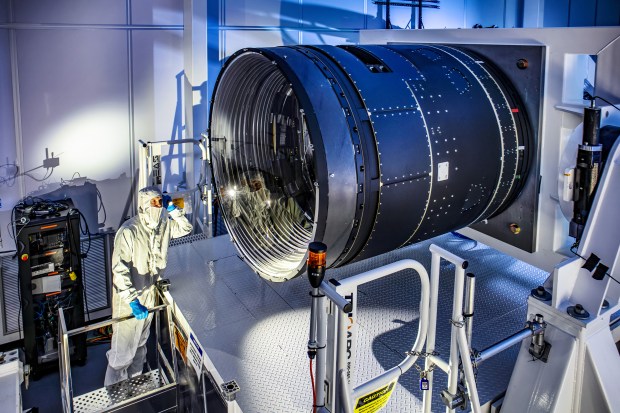
The largest digital camera ever made for astronomy is done
How to help astronomers study gamma-ray bursts.
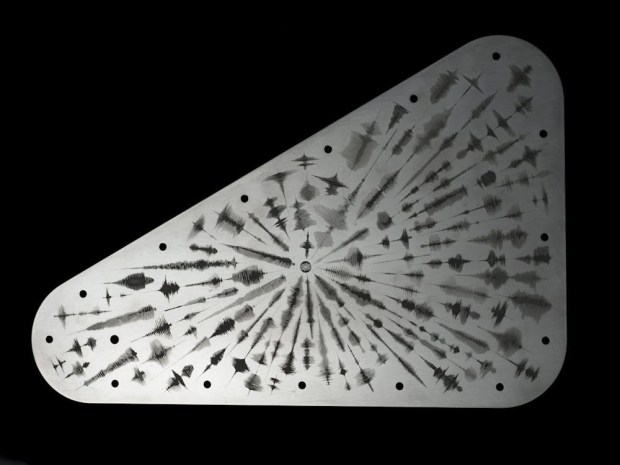
These are the clever messages headed to Jupiter aboard NASA’s Europa Clipper
Yes, an exploding star close to earth would make for a very bad day.
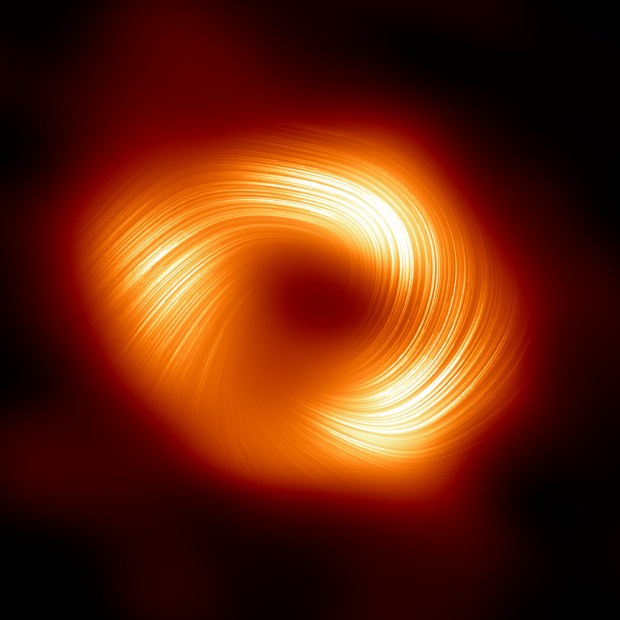
The Milky Way’s central black hole could have a hidden jet
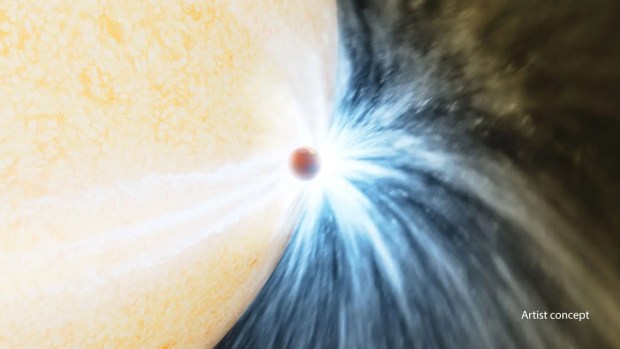
More than a few nearby stars show signs they ate young planets
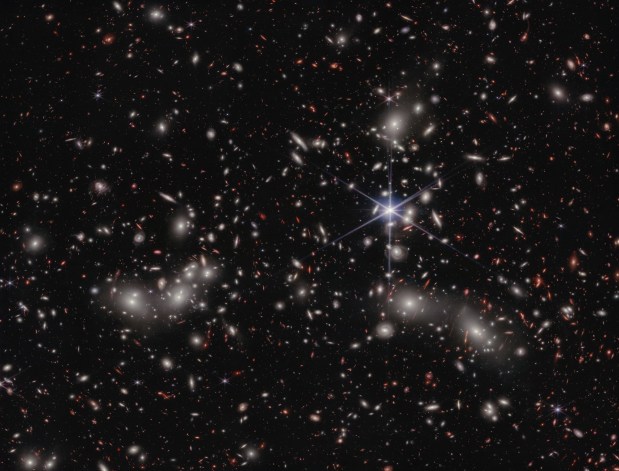
Dwarf galaxies turned on the lights near the dawn of time, JWST reveals
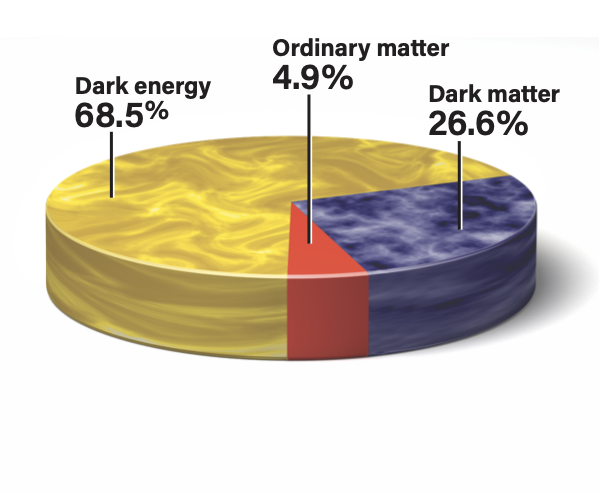
Are the percentages of dark matter and dark energy stable?
Science | July 12, 2022
NASA Releases First Breathtaking Images Taken by James Webb Space Telescope
The five pictures from the most powerful space observatory ever launched offer a deep look back in time and the promise of stellar things to come
:focal(800x602:801x603)/https://tf-cmsv2-smithsonianmag-media.s3.amazonaws.com/filer_public/9b/5a/9b5a39d6-7e7d-4065-bfd6-3b33ff97044d/deep-fieldsmacs0723_web.jpeg)
By Brian Handwerk
Science Correspondent
The deepest, sharpest infrared image ever captured of the distant universe was revealed last night—a stunning display of the galaxy cluster SMACS 0723 delivered by the recently launched James Webb Space Telescope. Just a century ago scientists believed there was only one galaxy, but this image reveals thousands—all found in a tiny speck of sky comparable in size to a single grain of sand held on a finger at arm’s length by someone standing on the ground. NASA Administrator Bill Nelson explained that images like this one, dubbed “Webb’s First Deep Field,” allow us to see the universe as it appeared far in the past—the light captured from these galaxies has been traveling through space for 4.6 billion years.
The wait for Webb wasn't quite that long. But finally, more than three decades after its conception and after six months in orbit, the James Webb Space Telescope ’s first full-color images are delivering an unprecedented look at our Universe. After the shot revealed last night by President Biden, scientists released four more amazing images today, the first of many incredible visuals to come.
/https://tf-cmsv2-smithsonianmag-media.s3.amazonaws.com/filer_public/d9/15/d9156480-7584-4703-916a-c0d7ee2b455c/deep-fieldsmacs0723_web.jpg)
The initial images include the Carina Nebula, a dynamic region of new star birth with at least a dozen massive stars 50 to 100 times the size of our own Sun, and the Southern Ring Nebula, a huge and expanding cloud of gas surrounding a star in its death throes. Webb also captured images of Stephan’s Quintet, a compact group of five galaxies found in the constellation Pegasus, and of the intriguing planet WASP-96b, a gassy giant some 1,150 light-years from Earth.
Astrophysicist John Mather, senior project scientist for the Webb Telescope and a Nobel Prize winner, began work on Webb back in 1995 just after he helped to measure the Big Bang and determine the age of the universe “It’s the next question,” he said as the images were revealed, “after you know how it started, what happened then?” The telescope, Mather is certain, will help provide some answers. “This is our time machine, and I’m so thrilled that we got a chance to do it.”
/https://tf-cmsv2-smithsonianmag-media.s3.amazonaws.com/filer_public/df/1a/df1ae8e3-2ccb-4410-a6e2-ca19ac6bb1ed/stsci-j-p22031a-4000pxcarinanebula_web.jpg)
The roughly $10 billion telescope allows us to see deeper into space, and farther back towards the origins of our universe, than humans have ever been able to see before. Webb can observe light from the universe’s very first stars and galaxies that formed 13.5 billion years ago, just a few hundred million years after the Big Bang, and track those stars and galaxies’ evolution throughout the entire age of the universe.
Though Webb will allow us to explore deeper into space than ever before, the telescope will do so much more . Webb will pull the curtain from nebulas to see where planetary systems and stars are born, which have previously been shielded from visible light observatories by dusty clouds. Infrared light observations allow Webb to peer through that dust to reveal the birth of stars, and even see through churning gasses to the black hole at the center of our own galaxy . The telescope will also offer clearer looks at exoplanets beyond our solar system, whose atmospheres are shrouded by clouds. That will enable scientists like Mercedes Lopez-Morales to study their composition and their atmospheres to see if any of them may be like Earth—and even if they might be suitable for life.
“Looking at the universe with Webb will be like looking at a familiar photo with a different set of glasses that allow us to see new details in that photo that we had never seen before,” says Lopez-Morales, an astrophysicist at the Center for Astrophysics, Harvard & Smithsonian. “Everywhere Webb will look, we will see something new.”
/https://tf-cmsv2-smithsonianmag-media.s3.amazonaws.com/filer_public/fd/9f/fd9f1dfe-20b5-4ea7-9a92-d882fa7ae763/exoplanetwasp96b_web.jpg)
Successor to the Hubble telescope, which is still operating after 30 years, the Webb is the most powerful and complex scientific observatory every built. The 25-year project, a joint effort of NASA, the European Space Agency (ESA) and the Canadian Space agency, endured numerous complications, including costs that ballooned from initial estimates of just $1 billion to $3.5 billion, a 2011 NASA budget cut, and delay after delay that left some wondering whether Webb would ever get off the ground.
But the fact that it’s now in orbit, and operational, stands as a testament to scientific collaboration on a massive scale. The awe-inspiring images Webb captures of our Universe, and the discoveries it promises about its origins and our own, are the culmination of work by some 1,200 scientists, technicians and engineers from 14 countries.
“Something like the Webb Telescope really shows what humans can do when we get together,” says Lopez-Morales. “When we put our mind to it and we persevere for years, and everybody works together, things like this can be done and to me that’s inspirational.”
/https://tf-cmsv2-smithsonianmag-media.s3.amazonaws.com/filer_public/28/4d/284dfc1c-6f39-4c8d-b46d-8b91f14f94b0/planetarynebulangc3132_web.jpg)
NASA Administrator James Webb oversaw many breakthroughs including the Apollo missions that landed the first humans on the moon, and explorations by America’s first interplanetary spacecraft. The telescope bearing his name—to which some scientists objected because of Webb’s acquiescence with his era’s homophobic government policies—launched on December 25, 2021, when an Ariane 5 rocket blasted off from Europe’s Spaceport in French Guiana. In space it carefully unfolded a tennis court-sized protective sunshield , and a honeycomb of 18 hexagonal, gold-lined mirrors more than 21 feet across, each aligned to 1/10,000th the width a single human hair. The mirrors allowed Webb to collect large amounts of light and thus see more of space than any other instrument when it settled into orbit almost one million miles above Earth.
Using sensor systems and four different cameras the telescope works in infrared light, a wavelength just beyond what we can see, and traces thermal radiation. Our own atmosphere blocks infrared light from space, which is one reason the Webb has to operate from orbit. The telescope must also be kept very cold, -364 degrees Fahrenheit, lest its own heat interfere with the sensors. That’s achieved by its location in deep, cold space and aided by that protective sun shield.
/https://tf-cmsv2-smithsonianmag-media.s3.amazonaws.com/filer_public/dc/5f/dc5f2446-740d-4dd5-8c21-1e960b5b60a6/stephansquintet_web.jpg)
The telescope, powered by a solar array, may be able to operate for 20 years or more. Unlike low-orbit Hubble, which can be serviced, Webb’s distance, orbiting beyond the Moon, means it has been designed to be self-sufficient during the life of the mission.
Webb’s to-do list is extremely impressive. Scientists are ready with well-laid plans to explore the early universe, chart how galaxies form and evolve over time, observe the lifecycles of stars, and delve into the mysteries of exoplanets. But the telescope’s greatest discoveries might not lie in any of these areas.
“It’s very likely that we will discover things that we cannot readily explain and had not thought about,” says Lopez-Morales. “That is how breakthroughs in human knowledge happen and so I am very excited to see what Webb’s observations will reveal to us.”
Get the latest Science stories in your inbox.
Brian Handwerk | READ MORE
Brian Handwerk is a science correspondent based in Amherst, New Hampshire.
Powerful observatories reveal 5 breathtaking corners of the universe hidden to human eyes (images)
There's a whole universe out there that we can't see — without a little help from our machines, that is.
Even if you were to theoretically travel to the darkest desert on Earth, wait until after sundown and peer up at the night sky, you wouldn't be able to see every star there is to see.
There'd be countless more scattered across the universe, hidden not just by distance but also because your eyes aren't built to perceive the signals they emit — unseeable signals like infrared light, radio waves and X-ray emissions. In fact, humans can only see a tiny fraction of the electromagnetic spectrum . It's a sliver known as the "visible light region."
But as Stephen Hawking once said , we brilliantly step past these limitations with "our minds and our machines" — and once again, our species has managed to live up to that phrase.
On Thursday (Sept. 14), scientists presented five new deep-space images captured in a variety of invisible-to-human wavelengths. It's a stunning collection of visuals that reveal some absolutely riveting corners of the cosmos. Each portrait is constructed with data collected by powerful telescopes, including NASA's Chandra X-ray Observatory , the now-retired Spitzer Space Telescope , the iconic James Webb Space Telescope and the Very Large Telescope (to name just some).
Basically, these instruments are able to capture outflows of non-visible light radiation emanating from distant regions of space in such a way that scientists can take that information, overlay as necessary and turn it into images we can admire.
Now that we know what we're looking at, let's go through the lot.
The first image NASA highlights in a statement about the five pieces is titled the "Galactic Center." Sitting about 26,000 light-years from Earth, this is literally the center of the Milky Way galaxy that we live in. It contains a supermassive black hole, superheated clouds of gas, neutron stars (which are stellar beings so dense a tablespoon of one would equal something like the weight of Mount Everest) and other trippy things.
Get the Space.com Newsletter
Breaking space news, the latest updates on rocket launches, skywatching events and more!
The reason it looks kind of blobby instead of swirly like you might imagine a galactic center to look is due to the fact that we are looking at it from within the galaxy. This internal perspective is actually one reason scientists with the Event Horizon Telescope Collaboration selected M87* , the black hole in one of our neighboring galaxies, as the subject of humanity's first black hole image instead of Sgr A* , the one in the center of the Milky Way. It was easier to inspect the center of a galaxy that we can see panoramically. (The EHT team did eventually manage to get an image of Sgr A*. That was humanity's second black hole image).
In this view of the Milky Way's core, Chandra data leads the charge, its observations seen in orange, green, blue and purple.
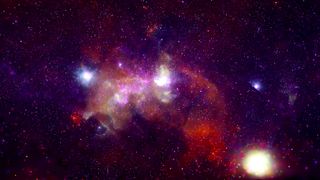
NASA then moves on to Kepler's Supernova Remnant , which the agency says represents the remains of a white dwarf that exploded after undergoing a thermonuclear explosion.
White dwarfs are the dying cores of stars that once thrived and shined the way our sun currently does. Someday, our sun will become a white dwarf as well.
In this image, Chandra data is seen in blue and shows a "powerful blast wave that ripped through space after the detonation," NASA explains, while infrared data from Spitzer is seen in red, and optical light from the Hubble Space Telescope is seen in cyan and yellow. The latter two show debris of the destroyed star.
To be clear, all the colors mentioned may not be easily discernible because of how scientists overlay certain bits of information. For instance, if you overlay yellow data with red data, you'd see more of an orange hue. If you'd like to see all the colors individually, however, here's a page on Chandra's website that shows you non-overlain images of the discussed portraits as well.
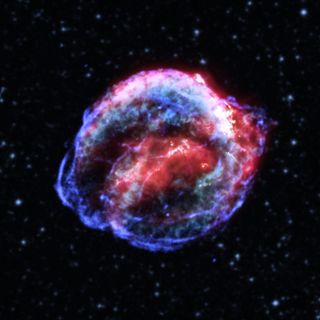
Next up, we have ESO 137-001, a galaxy that's moving through space at 1.5 million mph (2.4 million kph) while leaving two tails behind that are made of superheated gas.
Chandra observations capture this gas in blue, the VLT indicates hydrogen present in red and Hubble's optical and infrared data is shown in orange and cyan. (Yes, Hubble can collect infrared light like the James Webb Space Telescope, but the JWST is far better at it).
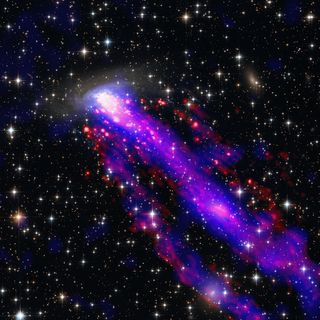
Keeping with the theme of galaxies, NASA then highlights the spiral galaxy NGC 1365 in a rather ghostly scene. This realm, according to the statement , contains a supermassive black hole that's being fed a steady stream of material.
Chandra observations, shown in purple to create that Barbie-esque glow, reveal some of that material yet to enter the void. The JWST's impressive infrared sensors had a hand in this visual as well, offering red, green and blue accents that are pretty difficult to see in this version.
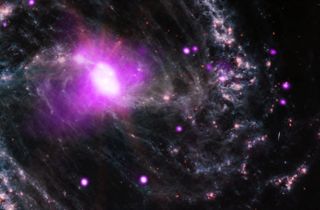
For the final of the so-called "Fab Five" images, scientists offer a dreamy view of the aftermath of a collapsed star. This is the Vela Pulsar, spied by NASA's Imaging X-ray Polarimetry Explorer (IXPE), Chandra and Hubble. These instruments' data are distributed in the light blue, purple and yellow, respectively.
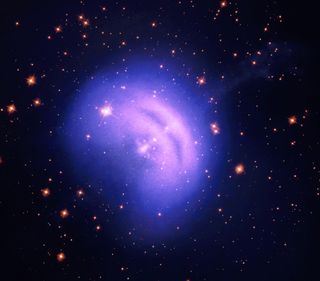
But despite the "Fab Five" title, it's worth noting that these images may as well be collectively called the "Fab Million." That does sound a bit weird, but what I mean to say is it's really hard not to focus on the absolute wealth of stars, galaxies and who knows what else that's present in the background of these photos.
Every single one of those sparkles represents a cosmic marvel just as beautiful as the one we're seeing up close — in the way that every image we take of someone in public tends to have people in the background with lives as rich as our subject's.
Day by day, each of those sparkles seems to be coming to the forefront, thanks to our minds and our machines.
Join our Space Forums to keep talking space on the latest missions, night sky and more! And if you have a news tip, correction or comment, let us know at: [email protected].
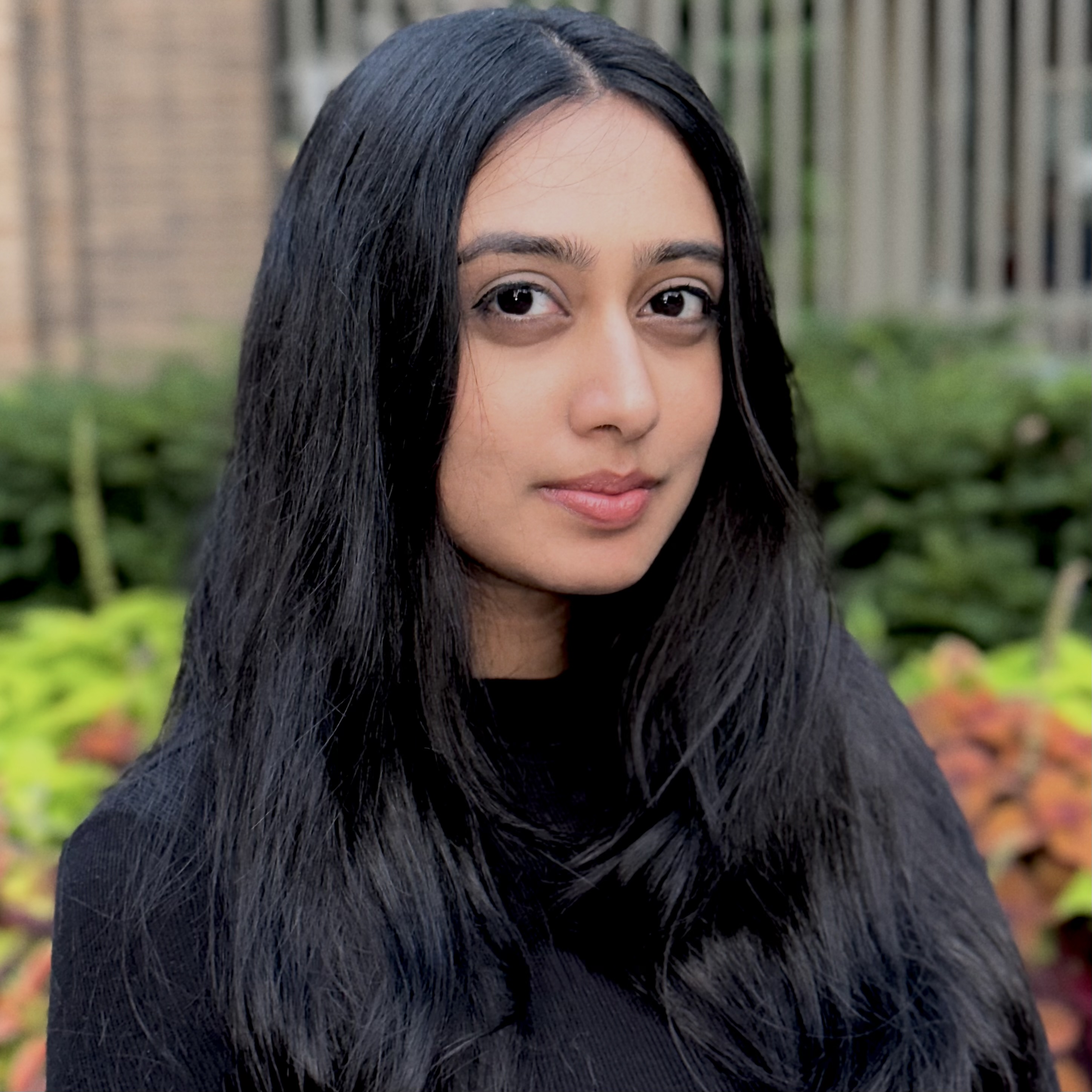
Monisha Ravisetti is Space.com's Astronomy Editor. She covers black holes, star explosions, gravitational waves, exoplanet discoveries and other enigmas hidden across the fabric of space and time. Previously, she was a science writer at CNET, and before that, reported for The Academic Times. Prior to becoming a writer, she was an immunology researcher at Weill Cornell Medical Center in New York. She graduated from New York University in 2018 with a B.A. in philosophy, physics and chemistry. She spends too much time playing online chess. Her favorite planet is Earth.
China's Chang'e 6 mission to collect samples of the far side of the moon enters lunar orbit (video)
China launches Chang'e 6 sample-return mission to moon's far side (video)
Scientists use XRISM spacecraft to predict fate of matter around monster supermassive black hole
- skynr13 i think your first comment in this article, "Even if you were to theoretically travel to the darkest desert on Earth, wait until after sundown and peer up at the night sky, you wouldn't be able to see every star there is to see. There'd be countless more scattered across the universe, hidden not just by distance but also because your eyes aren't built to perceive the signals they emit — unseeable signals like infrared light, radio waves and X-ray emissions." Besides the human eye not being able to see infrared light, radio waves and X-ray emissions, the main reason we can't see every star is the human eye just can't see that far. I think if we could see every star in the Universe just in visible light the night sky would be entirely white with light from every star being seen side by side. Reply
- billslugg The reason we don't see solid white is that there are not enough stars out there. There are only a finite number of stars visible. Some are hidden by dust, some are receding faster than c, and some of the light has not gotten here yet. Reply
- Classical Motion I'll bet you can take any and all dark areas, and with time exposure, all will be white. The absence of light can not be found in the night sky. We just don't have the sensitivity yet. I don't think one can find a direction that doesn't have a photon in it. Photons are the most numerous, the longest lasting and also the largest structures in the cosmos. Reply
- billslugg Yes, the Universe is bright in all directions, but not in visible light. It is bright at 3K. Reply
- Classical Motion I think there is a weak blanket of white light all thru space. Visible light. Star light. F spectrum wise, not eyeball sensitivity wise. Time can integrate it for us to see. And I'll bet in any direction. Just an opinion. I can't prove it. Reply
- skynr13 All you guys get the jist of what I was saying. Basically though, if a human eye could see every Star out to 13 billion light years, the glowing point of each star in every Galaxy would paste the sky so full of Stars you couldn't see beyond that to the darkness of Space. Reply
- View All 6 Comments
Most Popular
- 2 China's Chang'e 6 probe to the moon's far side has a big lunar mystery to solve
- 3 4 large incoming solar bursts could supercharge the auroras this weekend
- 4 Sierra Space's 1st Dream Chaser space plane aces key tests. Next stop: Florida launch site.
- 5 NASA's Nancy Grace Roman Telescope will hunt for tiny black holes left over from the Big Bang
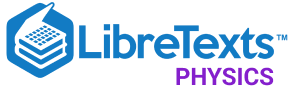
- school Campus Bookshelves
- menu_book Bookshelves
- perm_media Learning Objects
- login Login
- how_to_reg Request Instructor Account
- hub Instructor Commons
- Download Page (PDF)
- Download Full Book (PDF)
- Periodic Table
- Physics Constants
- Scientific Calculator
- Reference & Cite
- Tools expand_more
- Readability
selected template will load here
This action is not available.
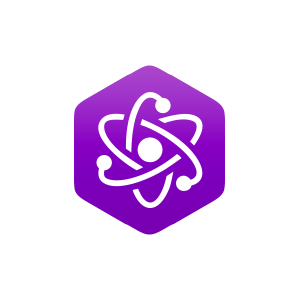
7.2: Overview of Our Planetary System
- Last updated
- Save as PDF
- Page ID 64692
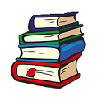
\( \newcommand{\vecs}[1]{\overset { \scriptstyle \rightharpoonup} {\mathbf{#1}} } \)
\( \newcommand{\vecd}[1]{\overset{-\!-\!\rightharpoonup}{\vphantom{a}\smash {#1}}} \)
\( \newcommand{\id}{\mathrm{id}}\) \( \newcommand{\Span}{\mathrm{span}}\)
( \newcommand{\kernel}{\mathrm{null}\,}\) \( \newcommand{\range}{\mathrm{range}\,}\)
\( \newcommand{\RealPart}{\mathrm{Re}}\) \( \newcommand{\ImaginaryPart}{\mathrm{Im}}\)
\( \newcommand{\Argument}{\mathrm{Arg}}\) \( \newcommand{\norm}[1]{\| #1 \|}\)
\( \newcommand{\inner}[2]{\langle #1, #2 \rangle}\)
\( \newcommand{\Span}{\mathrm{span}}\)
\( \newcommand{\id}{\mathrm{id}}\)
\( \newcommand{\kernel}{\mathrm{null}\,}\)
\( \newcommand{\range}{\mathrm{range}\,}\)
\( \newcommand{\RealPart}{\mathrm{Re}}\)
\( \newcommand{\ImaginaryPart}{\mathrm{Im}}\)
\( \newcommand{\Argument}{\mathrm{Arg}}\)
\( \newcommand{\norm}[1]{\| #1 \|}\)
\( \newcommand{\Span}{\mathrm{span}}\) \( \newcommand{\AA}{\unicode[.8,0]{x212B}}\)
\( \newcommand{\vectorA}[1]{\vec{#1}} % arrow\)
\( \newcommand{\vectorAt}[1]{\vec{\text{#1}}} % arrow\)
\( \newcommand{\vectorB}[1]{\overset { \scriptstyle \rightharpoonup} {\mathbf{#1}} } \)
\( \newcommand{\vectorC}[1]{\textbf{#1}} \)
\( \newcommand{\vectorD}[1]{\overrightarrow{#1}} \)
\( \newcommand{\vectorDt}[1]{\overrightarrow{\text{#1}}} \)
\( \newcommand{\vectE}[1]{\overset{-\!-\!\rightharpoonup}{\vphantom{a}\smash{\mathbf {#1}}}} \)
Learning Objectives
By the end of this section, you will be able to:
- Describe how the objects in our solar system are identified, explored, and characterized
- Describe the types of small bodies in our solar system, their locations, and how they formed
- Model the solar system with distances from everyday life to better comprehend distances in space
The solar system 1 consists of the Sun and many smaller objects: the planets, their moons and rings, and such “debris” as asteroids, comets, and dust. Decades of observation and spacecraft exploration have revealed that most of these objects formed together with the Sun about 4.5 billion years ago. They represent clumps of material that condensed from an enormous cloud of gas and dust. The central part of this cloud became the Sun, and a small fraction of the material in the outer parts eventually formed the other objects.
During the past 50 years, we have learned more about the solar system than anyone imagined before the space age. In addition to gathering information with powerful new telescopes, we have sent spacecraft directly to many members of the planetary system. (Planetary astronomy is the only branch of our science in which we can, at least vicariously, travel to the objects we want to study.) With evocative names such as Voyager , Pioneer , Curiosity , and Pathfinder , our robot explorers have flown past, orbited, or landed on every planet, returning images and data that have dazzled both astronomers and the public. In the process, we have also investigated two dwarf planets, hundreds of fascinating moons, four ring systems, a dozen asteroids, and several comets (smaller members of our solar system that we will discuss later).
Our probes have penetrated the atmosphere of Jupiter and landed on the surfaces of Venus, Mars, our Moon , Saturn’s moon Titan, the asteroids Eros, Itokawa, Ryugu, and Bennu, and the Comet Churyumov-Gerasimenko (usually referred to as 67P). Humans have set foot on the Moon and returned samples of its surface soil for laboratory analysis (Figure 7.2). We have flown a helicopter drone on Mars. We have even discovered other places in our solar system that might be able to support some kind of life.
Link to Learning
View this gallery of NASA images that trace the history of the Apollo mission.
An Inventory
The Sun, a star that is brighter than about 80% of the stars in the Galaxy, is by far the most massive member of the solar system, as shown in Table 7.1. It is an enormous ball about 1.4 million kilometers in diameter, with surface layers of incandescent gas and an interior temperature of millions of degrees. The Sun will be discussed in later chapters as our first, and best-studied, example of a star.
Table 7.1 also shows that most of the material of the planets is actually concentrated in the largest one, Jupiter , which is more massive than all the rest of the planets combined. Astronomers were able to determine the masses of the planets centuries ago using Kepler’s laws of planetary motion and Newton’s law of gravity to measure the planets’ gravitational effects on one another or on moons that orbit them (see Orbits and Gravity). Today, we make even more precise measurements of their masses by tracking their gravitational effects on the motion of spacecraft that pass near them.
Beside Earth, five other planets were known to the ancients—Mercury, Venus, Mars, Jupiter, and Saturn—and two were discovered after the invention of the telescope: Uranus and Neptune. The eight planets all revolve in the same direction around the Sun. They orbit in approximately the same plane, like cars traveling on concentric tracks on a giant, flat racecourse. Each planet stays in its own “traffic lane,” following a nearly circular orbit about the Sun and obeying the “traffic” laws discovered by Galileo, Kepler, and Newton. Besides these planets, we have also been discovering smaller worlds beyond Neptune that are called trans-Neptunian object s or TNOs (see Figure 7.3). The first to be found, in 1930, was Pluto , but others have been discovered during the twenty-first century. One of them, Eris , is about the same size as Pluto and has at least one moon (Pluto has five known moons.) The largest TNOs are also classed as dwarf planets, as is the largest asteroid, Ceres . (Dwarf planets will be discussed further in the chapter on Rings, Moons, and Pluto). To date, more than 2600 of these TNOs have been discovered, and one, called Arrokoth, was explored by the New Horizons spacecraft.
Each of the planets and dwarf planets also rotates (spins) about an axis running through it, and in most cases the direction of rotation is the same as the direction of revolution about the Sun. The exceptions are Venus , which rotates backward very slowly (that is, in a retrograde direction), and Uranus and Pluto , which also have strange rotations, each spinning about an axis tipped nearly on its side. We do not yet know the spin orientations of Eris, Haumea, and Makemake.
The four planets closest to the Sun (Mercury through Mars) are called the inner or terrestrial planets . Often, the Moon is also discussed as a part of this group, bringing the total of terrestrial objects to five. (We generally call Earth’s satellite “the Moon,” with a capital M, and the other satellites “moons,” with lowercase m’s.) The terrestrial planets are relatively small worlds, composed primarily of rock and metal. All of them have solid surfaces that bear the records of their geological history in the forms of craters, mountains, and volcanoes (Figure 7.4).
The next four planets (Jupiter through Neptune) are much larger and are composed primarily of lighter ices, liquids, and gases. We call these four the jovian planets (after “Jove,” another name for Jupiter in mythology) or giant planets —a name they richly deserve (Figure 7.5). About 1,300 Earths could fit inside Jupiter, for example. These planets do not have solid surfaces on which future explorers might land. They are more like vast, spherical oceans with much smaller, dense cores.
Near the outer edge of the system lies Pluto , which was the first of the distant icy worlds to be discovered beyond Neptune (Pluto was visited by a spacecraft, the NASA New Horizons mission, in 2015 [see Figure 7.6]). Table 7.2 summarizes some of the main facts about the planets.
Example 7.1: Comparing Densities
Let’s compare the densities of several members of the solar system. The density of an object equals its mass divided by its volume. The volume ( V ) of a sphere (like a planet) is calculated using the equation
V = 4 3 π R 3 V = 4 3 π R 3
where π (the Greek letter pi) has a value of approximately 3.14. Although planets are not perfect spheres, this equation works well enough. The masses and diameters of the planets are given in Table 7.2. For data on selected moons, see Appendix G. Let’s use Saturn’s moon Mimas as our example, with a mass of 4 × 10 19 4 × 10 19 kg and a diameter of approximately 400 km (radius, 200 km = 2 × 10 5 m 200 km = 2 × 10 5 m ).
The volume of Mimas is
4 3 × 3.14 × ( 2 × 10 5 m ) 3 = 3.3 × 10 16 m 3 . 4 3 × 3.14 × ( 2 × 10 5 m ) 3 = 3.3 × 10 16 m 3 .
Density is mass divided by volume:
4 × 10 19 kg 3.3 × 10 16 m 3 = 1.2 × 10 3 kg/m 3 . 4 × 10 19 kg 3.3 × 10 16 m 3 = 1.2 × 10 3 kg/m 3 .
Note that the density of water in these units is 1000 kg/m 3 , so Mimas must be made mainly of ice, not rock. (Note that the density of Mimas given in Appendix G is 1.2, but the units used there are different. In that table, we give density in units of g/cm 3 , for which the density of water equals 1. Can you show, by converting units, that 1 g/cm 3 is the same as 1000 kg/m 3 ?)
Exercise \(\PageIndex{1}\)
Calculate the average density of our own planet, Earth. Show your work. How does it compare to the density of an ice moon like Mimas? See Table 7.2 for data.
For a sphere, density = mass ( 4 3 π R 3 ) kg/m 3 . density = mass ( 4 3 π R 3 ) kg/m 3 . For Earth, then, density = 6 × 10 24 kg 4.2 × 2.6 × 10 20 m 3 = 5.5 × 10 3 kg/m 3 . density = 6 × 10 24 kg 4.2 × 2.6 × 10 20 m 3 = 5.5 × 10 3 kg/m 3 . This density is four to five times greater than Mimas’. In fact, Earth is the densest of the planets.
Learn more about NASA’s mission to Pluto and see high-resolution images of Pluto and its moon Charon.
Smaller Members of the Solar System
Most of the planets are accompanied by one or more moons; only Mercury and Venus move through space alone. There are more than 210 known moons orbiting planets and dwarf planets (see Appendix G for a listing of the larger ones), and undoubtedly many other small ones remain undiscovered. The largest of the moons are as big as small planets and just as interesting. In addition to our Moon, they include the four largest moons of Jupiter (called the Galilean moons, after their discoverer) and the largest moons of Saturn and Neptune (confusingly named Titan and Triton).
Each of the giant planets also has rings made up of countless small bodies ranging in size from mountains to mere grains of dust, all in orbit about the equator of the planet. The bright rings of Saturn are, by far, the easiest to see. They are among the most beautiful sights in the solar system (Figure 7.7). But, all four ring systems are interesting to scientists because of their complicated forms, influenced by the pull of the moons that also orbit these giant planets.
The solar system has many other less-conspicuous members. Another group is the asteroids , rocky bodies that orbit the Sun like miniature planets, mostly in the space between Mars and Jupiter (although some do cross the orbits of planets like Earth—see Figure 7.8). Most asteroids are remnants of the initial population of the solar system that existed before the planets themselves formed. Some of the smallest moons of the planets, such as the moons of Mars, are very likely captured asteroids.
Another class of small bodies is composed mostly of ice, made of frozen gases such as water, carbon dioxide, and carbon monoxide; these objects are called comets (see Figure 7.9). Comets also are remnants from the formation of the solar system, but they were formed and continue (with rare exceptions) to orbit the Sun in distant, cooler regions—stored in a sort of cosmic deep freeze. This is also the realm of the larger icy worlds, called dwarf planets.
Finally, there are countless grains of broken rock, which we call cosmic dust, scattered throughout the solar system. When these particles enter Earth’s atmosphere (as millions do each day) they burn up, producing a brief flash of light in the night sky known as a meteor (meteors are often referred to as shooting stars). Occasionally, some larger chunk of rocky or metallic material survives its passage through the atmosphere and lands on Earth. Any piece that strikes the ground is known as a meteorite . (You can see meteorites on display in many natural history museums and can sometimes even purchase pieces of them from gem and mineral dealers.)
Voyagers in Astronomy: Carl Sagan: Solar System Advocate
The best-known astronomer in the world during the 1970s and 1980s, Carl Sagan devoted most of his professional career to studying the planets and considerable energy to raising public awareness of what we can learn from exploring the solar system (see Figure 7.10). Born in Brooklyn, New York, in 1934, Sagan became interested in astronomy as a youngster; he also credits science fiction stories for sustaining his fascination with what’s out in the universe.
In the early 1960s, when many scientists still thought Venus might turn out to be a hospitable place, Sagan calculated that the thick atmosphere of Venus could act like a giant greenhouse, keeping the heat in and raising the temperature enormously. He showed that the seasonal changes astronomers had seen on Mars were caused, not by vegetation, but by wind-blown dust. He was a member of the scientific teams for many of the robotic missions that explored the solar system and was instrumental in getting NASA to put a message-bearing plaque aboard the Pioneer spacecraft, as well as audio-video records on the Voyager spacecraft—all of them destined to leave our solar system entirely and send these little bits of Earth technology out among the stars.
To encourage public interest and public support of planetary exploration, Sagan helped found The Planetary Society, now the largest space-interest organization in the world. He was a tireless and eloquent advocate of the need to study the solar system close-up and the value of learning about other worlds in order to take better care of our own.
Sagan simulated conditions on early Earth to demonstrate how some of life’s fundamental building blocks might have formed from the “primordial soup” of natural compounds on our planet. In addition, he and his colleagues developed computer models showing the consequences of nuclear war for Earth would be even more devastating than anyone had thought (this is now called the nuclear winter hypothesis) and demonstrating some of the serious consequences of continued pollution of our atmosphere.
Sagan was perhaps best known, however, as a brilliant popularizer of astronomy and the author of many books on science, including the best-selling Cosmos , and several evocative tributes to solar system exploration such as The Cosmic Connection and Pale Blue Dot . His book The Demon Haunted World , completed just before his death in 1996, is perhaps the best antidote to fuzzy thinking about pseudo-science and irrationality in print today. An intriguing science fiction novel he wrote, titled Contact , which became a successful film as well, is still recommended by many science instructors as a scenario for making contact with life elsewhere that is much more reasonable than most science fiction.
Sagan was a master, too, of the television medium. His 13-part public television series, Cosmos , was seen by an estimated 500 million people in 60 countries and has become one of the most-watched series in the history of public broadcasting. A few astronomers scoffed at a scientist who spent so much time in the public eye, but it is probably fair to say that Sagan’s enthusiasm and skill as an explainer won more friends for the science of astronomy than anyone or anything else in the second half of the twentieth century.
In the two decades since Sagan’s death, no other scientist has achieved the same level of public recognition. Perhaps closest is the director of the Hayden Planetarium, Neil deGrasse Tyson , who followed in Sagan’s footsteps by making an updated version of the Cosmos program in 2014. Tyson is quick to point out that Sagan was his inspiration to become a scientist, telling how Sagan invited him to visit for a day at Cornell when he was a high school student looking for a career. However, the media environment has fragmented a great deal since Sagan’s time. It is interesting to speculate whether Sagan could have adapted his communication style to the world of cable television, Twitter, Facebook, and podcasts.
Two imaginative videos provide a tour of the solar system objects we have been discussing. Shane Gellert’s I Need Some Space uses NASA photography and models to show the various worlds with which we share our system. In the more science fiction-oriented Wanderers video, we see some of the planets and moons as tourist destinations for future explorers, with commentary taken from recordings by Carl Sagan.
A Scale Model of the Solar System
Astronomy often deals with dimensions and distances that far exceed our ordinary experience. What does 1.4 billion kilometers—the distance from the Sun to Saturn—really mean to anyone? It can be helpful to visualize such large systems in terms of a scale model.
In our imaginations, let us build a scale model of the solar system, adopting a scale factor of 1 billion (10 9 )—that is, reducing the actual solar system by dividing every dimension by a factor of 10 9 . Earth, then, has a diameter of 1.3 centimeters, about the size of a grape. The Moon is a pea orbiting this at a distance of 40 centimeters, or a little more than a foot away. The Earth-Moon system fits into a standard backpack.
In this model, the Sun is nearly 1.5 meters in diameter, about the average height of an adult, and our Earth is at a distance of 150 meters—about one city block—from the Sun. Jupiter is five blocks away from the Sun, and its diameter is 15 centimeters, about the size of a very large grapefruit. Saturn is 10 blocks from the Sun; Uranus, 20 blocks; and Neptune, 30 blocks. Pluto, with a distance that varies quite a bit during its 249-year orbit, is currently just beyond 30 blocks and getting farther with time. Most of the moons of the outer solar system are the sizes of various kinds of seeds orbiting the grapefruit, oranges, and lemons that represent the outer planets.
In our scale model, a human is reduced to the dimensions of a single atom, and cars and spacecraft to the size of molecules. Sending the Voyager spacecraft to Neptune involves navigating a single molecule from the Earth–grape toward a lemon 5 kilometers away with an accuracy equivalent to the width of a thread in a spider’s web.
If that model represents the solar system, where would the nearest stars be? If we keep the same scale, the closest stars would be tens of thousands of kilometers away. If you built this scale model in the city where you live, you would have to place the representations of these stars on the other side of Earth or beyond.
By the way, model solar systems like the one we just presented have been built in cities throughout the world. In Sweden, for example, Stockholm’s huge Globe Arena has become a model for the Sun, and Pluto is represented by a 12-centimeter sculpture in the small town of Delsbo, 300 kilometers away. Another model solar system is in Washington on the Mall between the White House and Congress (perhaps proving they are worlds apart?).
This model of the solar system shows all orbits and sizes to scale, and it lets you fly between the planets at an enhanced speed.
Making Connections: Names in the Solar System
We humans just don’t feel comfortable until something has a name. Types of butterflies, new elements, and the mountains of Venus all need names for us to feel we are acquainted with them. How do we give names to objects and features in the solar system?
Planets and moons are named after gods and heroes in Greek and Roman mythology (with a few exceptions among the moons of Uranus, which have names drawn from English literature). When William Herschel, a German immigrant to England, first discovered the planet we now call Uranus, he wanted to name it Georgium Sidus (George’s star) after King George III of his adopted country. This caused such an outcry among astronomers in other nations, however, that the classic tradition was upheld—and has been maintained ever since. Luckily, there were a lot of minor gods in the ancient pantheon, so plenty of names are left for the many small moons we are discovering around the giant planets. (Appendix G lists the larger moons). More recently, the names of dwarf planets and their moons have been drawn from the mythology of other cultures besides Greek and Roman.
Comets are often named after their discoverers (offering an extra incentive to comet hunters). Asteroids are named by their discoverers after just about anyone or anything they want. Recently, asteroid names have been used to recognize people who have made significant contributions to astronomy, including the three senior authors of this book.
That was pretty much all the naming that was needed while our study of the solar system was confined to Earth. But now, our spacecraft have surveyed and photographed many worlds in great detail, and each world has a host of features that also need names. To make sure that naming things in space remains multinational, rational, and somewhat dignified, astronomers have given the responsibility of approving names to a special committee of the International Astronomical Union (IAU), the body that includes scientists from every country that does astronomy.
This IAU committee has developed a set of rules for naming features on other worlds. For example, craters on Venus are named for women who have made significant contributions to human knowledge and welfare. Volcanic features on Jupiter’s moon Io, which is in a constant state of volcanic activity, are named after gods of fire and thunder from the mythologies of many cultures. Craters on Mercury commemorate famous novelists, playwrights, artists, and composers. On Saturn’s moon Tethys, all the features are named after characters and places in Homer’s great epic poem, The Odyssey . As we explore further, it may well turn out that more places in the solar system need names than Earth history can provide. Perhaps by then, explorers and settlers on these worlds will be ready to develop their own names for the places they may (if but for a while) call home.
You may be surprised to know that the meaning of the word planet has recently become controversial because we have discovered many other planetary systems that don’t look very much like our own. Even within our solar system, the planets differ greatly in size and chemical properties. The biggest dispute concerns Pluto, which is much smaller than the other eight major planets. The category of dwarf planet was invented to include Pluto and similar icy objects beyond Neptune. But is a dwarf planet also a planet? Logically, it should be, but even this simple issue of grammar has been the subject of heated debate among both astronomers and the general public.
- 1 The generic term for a group of planets and other bodies circling a star is planetary system . Ours is called the solar system because our Sun is sometimes called Sol . Strictly speaking, then, there is only one solar system; planets orbiting other stars are in planetary systems.
- 2 An AU (or astronomical unit) is the distance from Earth to the Sun.
- 3 We give densities in units where the density of water is 1 g/cm 3 . To get densities in units of kg/m 3 , multiply the given value by 1000.
Since the beginning of our history, humans have looked up at the sky and marveled at what they saw. The changing face of the Moon, the brilliance of the Sun, the movement of the planets, all of these and more awed our ancestors. In an attempt to understand, they created stories and prescribed meanings to the objects that filled the sky. Some also created elaborate models to reproduce the motion of objects in the heavens.
Today, scientists understand more about these celestial bodies. Some are unbelievably large, while others are smaller than a particle of dust. In this book, we will be exploring “small bodies” found in our solar system. Our solar system consists of one star, the Sun, and all the objects currently held in orbit by the gravity of our star. The solar system is just one part of a much, much larger group of stars that form the Milky Way Galaxy.
So what is a small body?
A small body is an object in space such as an asteroid or comet. Interplanetary dust, Kuiper Belt Objects, material in the Oort Cloud, planetary satellites and, yes, even Pluto and other dwarf planets can be considered “small bodies.”
The Oort Cloud is a collection of small bodies at the far reaches of our solar system (Tactile 1) . These objects are distributed in every direction and are not constrained to the primary orbital plane on which we find most planets, comets and asteroids. Countless icy bodies not confined to the ecliptic, or the plane on which Earth orbits, are found at vast distances from the Sun. The Oort Cloud is thought to be the birthplace of many long-period comets who rarely, if ever, get pulled in towards the inner solar system only to be flung back out towards the Oort Cloud due to the gravity of the Sun. These icy bodies are part of the family of “small bodies” in our solar system. As a whole, if the inner region of the Oort Cloud (about 2000 Astronomical Units, or AU from the Sun) were the size of a basketball (12” in diameter), the entire solar system from the Sun out to the orbit of Neptune would be about the size of a BB (3/16” in diameter)! The entire Oort Cloud extends from about 2000 AU to over 67,000 AU!
Note: An AU is a unit of distance and stands for an Astronomical Unit, which is the average distance from the Earth to the Sun and is about 150,000,000 km. Therefore, to say that Jupiter is found at 5 AUs means that it is about 5 times farther than the average distance from the Earth to the Sun. In miles, an AU is about 93,000,000 mi.
Kuiper Belt
The region extending outwards from Neptune’s orbit and generally in the same plane of the planets also contains many small bodies including those of the short-period comets, or those comets that take less than 200 years to make one orbit. This region is referred to as the Kuiper Belt and extends roughly 30 to 50 AU; although some suspected Kuiper Belt objects are found much further than 50 AU.
The New Horizons spacecraft will fly by Kuiper belt object, 2014 MU69, on December 31st 2018. Images from groundbased telescopes, Hubble telescope and the NASA Startospheric Observatory for Infrared Astronomy (SOFIA) indicate that MU 69 is an elongated object and may have two lobes or a binary shape. Stay tuned!
What is a comet?
The Oort Cloud and Kuiper Belt seem to be the birthplace of comets, therefore it is important to learn what these small bodies called comets are. A comet is a ball of ice and gas mixed with dust and rock that orbits the Sun. They date back to when the solar system was formed. Comets are often called “dirty snowballs” because they consist of a mixture of ice, rock and dust fragments.
A solid surface made of ice, rock and dust forms the nucleus of a comet. Tactile 2 details the nucleus of a comet discovered in 1969 called Comet 67P/Churyumov–Gerasimenko. The nucleus has two distinct lobes suggesting it may be the result of the collision of 2 comets in the past; again highlighting the dynamic nature of small bodies! The “mountains” on the right edge of the nucleus are 100’s of meters high. Note also the outgassing occurring near the upper center of the core. As a comet is “pulled” into the inner solar system by the gravity of the Sun, the solar radiation pressure and increased temperature cause the material in the nucleus to vaporize, releasing gas and dust formerly frozen in the nucleus. When that happens, those on Earth are sometimes able to see the coma and the tail as detailed in Tactile 3 . The coma is simply the gas and dust illuminated by the Sun directly surrounding the nucleus. On this tactile, the nucleus is found as a raised area towards the bottom of the coma and is labled to the left and probably has similar structures to the one explored on Tactile 2. The nucleus is, however, much much smaller than the coma or other features of a comet. Because of the outward radiating pressure from the Sun called solar wind, some of the material in the coma is “blown” away from the comet forming the tail. Here is where an interesting twist enters the story: The dust and gas are affected differently by the solar radiation. The more dense and massive dust is less affected by the solar radiation and retains more of a curved path due to the orbiting nucleus. The less dense gas is easily carried away from the nucleus and thus trails straight out away from the nucleus pointing directly away from the Sun. This is illustrated in Tactile 3 which shows the anatomy of a comet as it nears our Sun.
What are asteroids?
Although the distinction between comets and asteroids is not always easy to make, asteroids tend to be small rocky or metallic bodies that orbit the Sun mostly between Mars and Jupiter in what is called the Asteroid belt. Tactile 4 (not to scale by size or by distance) illustrates the location of the asteroid belt in relation to the other planets. Asteroids can be as big as 1000 km (600 miles) in diameter down to the size of small pebbles. Although they orbit the Sun, most are too small to be considered planets. The larger asteroids, on the other hand, may be classified as dwarf planets. They are not considered planets as they have not cleared the area around them of surrounding debris.
Tactile 5 highlights the asteroid Vesta, which has a diameter of 530 km. Vesta is unlike most asteroids in that it has different layers in its interior. These are exposed in the three “snowman” craters that can be found towards the top of the tactile and to the left of the ridges and valleys. The asteroid Vesta dispels the myth that all asteroids are uniform hunks of rock.
Asteroids are thought to be leftover material from the formation of our Solar System. They are classified into three major types: C, S, and X depending upon the abundance of rocky, metallic or clay like material.
Debris from comets and asteroids can often enter the Earth’s atmosphere and sometimes even collide with Earth. Smaller debris from comets and asteroids are often referred to as meteoroids. The terms “meteoroid,” “meteorite,” and “meteor” are often used interchangeably, but scientists define these using three different terms. Meteoroids are small parts of an asteroid or comet that are orbiting the Sun. When a meteoroid enters Earth’s atmosphere it is called a meteor and will usually vaporize and streak across the sky. Meteors are often called “shooting stars” or “falling stars” but have nothing to do with distant stars.
“Meteorites” are pieces of space debris that enter Earth’s atmosphere and stay intact to hit the Earth. They are primarily pieces of asteroids. They can be as small as a grain of rice or as large as a boulder. Most meteors and meteorites are categorized as either iron (mainly made of iron), stony (mainly made of minerals other than iron), or stony-iron (composed of both).
Asteroids, comets and meteoroids routinely hit the Earth. However, most of these are small and hardly noticeable. The larger the object, the more dangerous it is to the Earth.
If an asteroid more than 400 meters (a quarter-mile) wide hit the Earth, it could cause a global disaster such as the one that resulted in the extinction of the dinosaurs. However, these are extremely rare. Smaller asteroids or comets are still hazardous because, depending on their speed, angle of impact, and size, they can cause injuries, destroy cities, or cause deadly tsunamis. Scientists from NASA’s Near Earth Object program continually scan and track the motion of many of these objects, to proactively mitigate any potential collision issues with Earth.
Aside from a potential threat, asteroids are also a possible source for natural resources. Many of Earth’s metals and minerals are seriously depleted. Mining asteroids could help with this problem in the near future.
Unlike comets, most large asteroids are found in relatively stable orbits around the Sun and do not intersect with Earth’s orbit. One of these asteroids, Ceres (Tactile 6) is the largest known asteroid and can be referred to as a dwarf planet. It also sports curious features similar to those found on comets and also hints at a place of origin in the Kuiper belt. Notice the bumps in a crater at the center of Tactile 6 . These bright spots are referred to as “Spot 5” and were discovered by the Dawn spacecraft in 2015. They are found in a crater about 80 km wide and are thought to be brighter than the surrounding region as they contain a type of salt that probably originated from salty water erupting onto the surface and subsequent evaporation, hinting at recent geologic activity. Material has also been observed, both directly and indirectly, erupting onto the surface of Earth, Jupiter’s moon Io, and Saturn’s moon Enceladus.
Dwarf Planets
As the name implies, these planets are small bodies and smaller than the 8 known “major” planets in our solar system. From the Sun outward, the “major” planets include Mercury, Venus, Earth, Mars, Jupiter, Saturn, Uranus and Neptune and are labeled on Tactile 4 which includes the location of the asteroid belt (astr). Pluto, which was once classified as a major planet, resides in the outermost region of our solar system but it does not adequately fulfill all the requirements for maintaining major planet status.
The International Astronomical Union is the only recognized body that can officially name objects in space. They have determined that planets must:
- Orbit around the Sun
- Have sufficient mass for it to take on a nearly round shape, and
- Clear the neighborhood around its orbit
Many Dwarf Planets, on the other hand, are much smaller and fail to meet the 3rd criteria of clearing their neighborhood around its orbit. Pluto (Tactile 7) , for example, orbits the Sun and has sufficient mass for it to take on a nearly round shape, however, it has not cleared its neighborhood around its orbit. In other words, it is not massive enough to either capture other objects in its orbit, or to fling them out of its orbit due to gravitational forces. Therefore, Pluto and other objects meeting a similar fate are considered Dwarf Planets. However, let’s be clear that the demotion of Pluto to dwarf planet status has not altered Pluto itself or its significance in our solar system or the fascinating science yet to be discovered about this object. Tactile 7 is based off recent data acquired from the New Horizons mission and highlights some of the dynamic terrain found on its surface. Can you find the heart shped feature located at the bottom of the tactile?
Other named dwarf planets include Ceres, Haumea, Makemake and Eris; but many other yet to be discovered objects may meet the criteria of being considered dwarf planets.
Other small bodies in our solar system comprise what we call satellites. Satellites are most often thought of as “moons” orbiting around planets. However, the term can also refer to pieces of asteroids or comets, which orbit around other small objects. Note that as used here, the term ‘satellites’ refers to natural satellites and does not refer to the man-made variety, or spacecraft!
Our solar system is filled with intriguing satellites, some of which may even harbor oceans! Mercury and Venus have no satellites. The Earth has one satellite, our Moon. The Moon orbits around the Earth taking a little over 27 days to complete one revolution with respect to the background stars. Mars has two satellites named after the Greek characters Phobos and Deimos. Tactile 8 details the satellites of Mars to scale with each other and to scale by distance from Mars. Notice the large crater on the lower right of Phobos. Notice also the odd shape of Deimos. What would it be like to have 2 moons orbting Earth?
The larger gas giant planets of Jupiter, Saturn, Uranus and Neptune harbor a wide variety of satellites waiting to be investigated by future scientists and explorers. Will you be one of them?
As a whole, small bodies in our solar system provide a window into what the early solar system may have looked like and the materials that formed it. Furthermore, the continued study of small bodies in our solar system will benefit humans by providing better methods for protecting our own planet and providing possible sources of resources in the future.
We want to express our gratitude to Joe Lang, Operations Manager of Connoisseur Media of Erie, Pennsylvania who donated his time and talents for recording and editing the audio files for "Getting a Feel for Lunar Craters," "Understanding Small Worlds," and "Mars Science Laboratory" books.
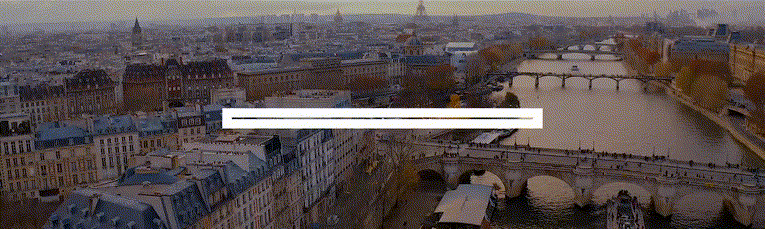
IMAGES
VIDEO
COMMENTS
The Crossword Solver found 30 answers to "of countless small bodies traveling through space", 6 letters crossword clue. The Crossword Solver finds answers to classic crosswords and cryptic crossword puzzles. Enter the length or pattern for better results. Click the answer to find similar crossword clues . Enter a Crossword Clue. Sort by Length.
This gateway is a region of space where objects called centaurs — small, icy bodies that orbit between Jupiter and Neptune — start to nudge closer to the sun. As they do so, they heat up and ...
NASA's Big Plans to Explore Small Bodies. Asteroids have been orbiting the sun for thousands of millennia in deep space, standing as ancient storytellers, holding clues about the formation of the solar system. NASA's first mission to collect a sample from an asteroid, the Origins, Spectral Interpretation, Resource Identification, Security ...
Most comets are thought to originate in the outermost parts of the solar system, the Kuiper belt and the much more distant Oort cloud. Each of these consists of countless small icy bodies that orbit the Sun. The farthest reaches of the Oort cloud extend perhaps to 100,000 AU, or some 9.3 trillion miles (15 trillion kilometers), from the Sun.
Furthering NASA's mission to extend human presence into the solar system, missions investigating near Earth asteroids enable exploration of deep space and are also a stepping stone to longer, more extensive missions to Mars and other planetary bodies. Historically, missions to small bodies have been fly-by trajectories, but recent missions ...
The solar system [1] consists of the Sun and many smaller objects: the planets, their moons and rings, and such "debris" as asteroids, comets, and dust. Decades of observation and spacecraft exploration have revealed that most of these objects formed together with the Sun about 4.5 billion years ago. They represent clumps of material that ...
Other small bodies travel in unstable orbits that cross the paths of one or more of the planets. Those include (1) most observed comets, (2) near-Earth asteroids, most with orbits that cross either Earth's orbit or Mars's orbit and some with orbits that lie mostly or entirely inside Earth's orbit and cross the orbits of Venus or of both Venus and Mercury while closely approaching Earth ...
Describe the types of small bodies in our solar system, their locations, and how they formed; Model the solar system with distances from everyday life to better comprehend distances in space; The solar system 1 consists of the Sun and many smaller objects: the planets, their moons and rings, and such "debris" as asteroids, comets, and dust ...
Scientists want to know whether any changes to the human body induced by long-duration space travel come with indicators that can also be detected in blood and urine. Astronauts will: Provide blood and urine samples before, during, and after spaceflight, and. Complete questionnaires regarding health and exercise habits.
Describe the types of small bodies in our solar system, their locations, and how they formed; Model the solar system with distances from everyday life to better comprehend distances in space; The solar system 1 consists of the Sun and many smaller objects: the planets, their moons and rings, and such "debris" as asteroids, comets, and dust ...
Countless small groups of galaxies are even farther away, and if we travel some 55 million light-years, we get to the center of the largest cluster of galaxies in our cosmic space, the Virgo ...
SmlBodies_factsheet.ai. National Aeronautics and Space Administration. Big Discoveries! Our solar system was born from a wispy cloud of gas and dust. Some. researchers believe that a shockwave from a nearby supernova explosion caused the cloud to compress and collapse in on itself, pulling enough material toward its center to create a star.
NASA Administrator Bill Nelson explained that images like this one, dubbed "Webb's First Deep Field," allow us to see the universe as it appeared far in the past—the light captured from ...
Next up, we have ESO 137-001, a galaxy that's moving through space at 1.5 million mph (2.4 million kph) while leaving two tails behind that are made of superheated gas.
Each of the giant planets also has rings made up of countless small bodies ranging in size from mountains to mere grains of dust, all in orbit about the equator of the planet. The bright rings of Saturn are, by far, the easiest to see. They are among the most beautiful sights in the solar system (Figure 7.7).
Answers for Small body travelling through space (6) crossword clue, 6 letters. Search for crossword clues found in the Daily Celebrity, NY Times, Daily Mirror, Telegraph and major publications. Find clues for Small body travelling through space (6) or most any crossword answer or clues for crossword answers.
As the name implies, these planets are small bodies and smaller than the 8 known "major" planets in our solar system. From the Sun outward, the "major" planets include Mercury, Venus, Earth, Mars, Jupiter, Saturn, Uranus and Neptune and are labeled on Tactile 4 which includes the location of the asteroid belt (astr).
1. What is the name of small bodies (smaller than asteroids) that travel through space? _____ 2. Are most of these bodies smaller than pebbles or bigger than boulders? _____ 3. Where do most of these objects come from? _____ 4. What is the name of these same small bodies as they fall through the Earth's atmosphere? _____ 5.
In fact, it turns out that gravity is nothing more than curved space, or, more specifically, the curvature or warpage of four- dimensional space-time. A simple analogy might help us to understand this notoriously hard-to-visualize concept. If a group of ants spend their entire lives on the essentially 2- dimensional surface of a trampoline, and ...
Answers for of countless small bodies moving through space (8) crossword clue, 8 letters. Search for crossword clues found in the Daily Celebrity, NY Times, Daily Mirror, Telegraph and major publications. Find clues for of countless small bodies moving through space (8) or most any crossword answer or clues for crossword answers.