Appointments at Mayo Clinic
Sundowning: late-day confusion, i've heard that sundowning may happen with dementia. what is sundowning and how is it treated.
The term "sundowning" refers to a state of confusion that occurs in the late afternoon and lasts into the night. Sundowning can cause various behaviors, such as confusion, anxiety, aggression or ignoring directions. Sundowning also can lead to pacing or wandering.
Sundowning isn't a disease. It's a group of symptoms that occurs at a specific time of the day. These symptoms may affect people with Alzheimer's disease and other types of dementia. The exact cause of sundowning is not known.
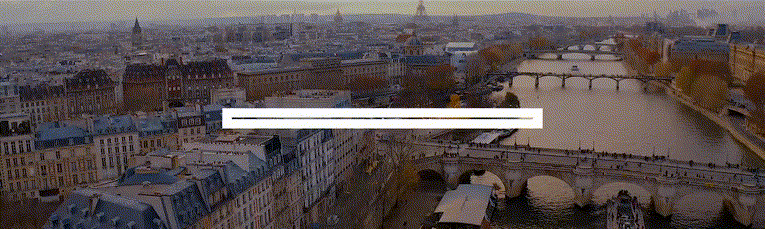
Factors that may worsen late-day confusion
- Spending a day in a place that's not familiar.
- Low lighting.
- Increased shadows.
- Disruption of the body's "internal clock."
- Trouble separating reality from dreams.
- Being hungry or thirsty.
- Presence of an infection, such as a urinary tract infection.
- Being bored or in pain.
- Depression.
Tips for reducing sundowning
- Keep a predictable routine for bedtime, waking, meals and activities.
- Plan for activities and exposure to light during the day to support nighttime sleepiness.
- Limit daytime napping.
- Limit caffeine and sugar to morning hours.
- Turn on a night light to reduce agitation that occurs when surroundings are dark or not familiar.
- In the evening, try to reduce background noise and stimulating activities. This includes TV viewing, which can sometimes be upsetting.
- In a strange or not familiar setting, bring familiar items, such as photographs. They can create a more relaxed setting.
- In the evening, play familiar, gentle music or relaxing sounds of nature, such as the sound of waves.
Some research suggests that a low dose of melatonin may help ease sundowning. Melatonin is a naturally occurring hormone that induces sleepiness. It can help when taken alone or in combination with exposure to bright light during the day.
It's possible that a medicine side effect, pain, depression or other condition could contribute to sundowning. Talk with a healthcare professional if you suspect that a condition might be making someone's sundowning worse. A urinary tract infection or sleep apnea could be contributing to sundowning, especially if it comes on quickly.
Jonathan Graff-Radford, M.D.
There is a problem with information submitted for this request. Review/update the information highlighted below and resubmit the form.
From Mayo Clinic to your inbox
Sign up for free and stay up to date on research advancements, health tips, current health topics, and expertise on managing health. Click here for an email preview.
Error Email field is required
Error Include a valid email address
To provide you with the most relevant and helpful information, and understand which information is beneficial, we may combine your email and website usage information with other information we have about you. If you are a Mayo Clinic patient, this could include protected health information. If we combine this information with your protected health information, we will treat all of that information as protected health information and will only use or disclose that information as set forth in our notice of privacy practices. You may opt-out of email communications at any time by clicking on the unsubscribe link in the e-mail.
Thank you for subscribing!
You'll soon start receiving the latest Mayo Clinic health information you requested in your inbox.
Sorry something went wrong with your subscription
Please, try again in a couple of minutes
- Alzheimer's prevention: Does it exist?
- Todd WD. Potential pathways for circadian dysfunction and sundowning-related behavioral aggression in Alzheimer's disease and related dementia. Frontiers in Neuroscience. 2020; doi:10.3389/fnins.2020.00910.
- Sleep issues and sundowning. Alzheimer's Association. http://www.alz.org/care/alzheimers-dementia-sleep-issues-sundowning.asp. Accessed April 4, 2022.
- Managing personality and behavior changes in Alzheimer's. National Institute on Aging. https://www.nia.nih.gov/health/managing-personality-and-behavior-changes-alzheimers. Accessed April 4, 2022.
- Francis J. Delirium and confusional states: Prevention, treatment, and prognosis. http://www.uptodate.com/home. Accessed April 4, 2022.
- Graff-Radford J (expert opinion). Mayo Clinic. April 7, 2022.
- Tips for coping with sundowning. National Institute on Aging. https://www.nia.nih.gov/health/tips-coping-sundowning. Accessed April 4, 2022.
- Reiter RJ, et al. Brain washing and neural health: Role of age, sleep and the cerebrospinal fluid melatonin rhythm. Cellular and Molecular Life Sciences. 2023; doi:10.1007/s00018-023-04736-5.
Products and Services
- Assortment of Products for Independent Living from Mayo Clinic Store
- A Book: Mayo Clinic on Alzheimer's Disease
- A Book: Day to Day: Living With Dementia
- A Book: Mayo Clinic on Healthy Aging
- Give today to find Alzheimer's cures for tomorrow
- Alzheimer's sleep problems
- Alzheimer's: New treatments
- Alzheimer's 101
- Understanding the difference between dementia types
- Alzheimer's disease
- Alzheimer's genes
- Alzheimer's drugs
- Alzheimer's stages
- Antidepressant withdrawal: Is there such a thing?
- Antidepressants and alcohol: What's the concern?
- Antidepressants and weight gain: What causes it?
- Antidepressants: Can they stop working?
- Antidepressants: Side effects
- Antidepressants: Selecting one that's right for you
- Antidepressants: Which cause the fewest sexual side effects?
- Anxiety disorders
- Atypical antidepressants
- Caregiver stress
- Clinical depression: What does that mean?
- Corticobasal degeneration (corticobasal syndrome)
- Depression and anxiety: Can I have both?
- Depression, anxiety and exercise
- What is depression? A Mayo Clinic expert explains.
- Depression in women: Understanding the gender gap
- Depression (major depressive disorder)
- Depression: Supporting a family member or friend
- Diagnosing Alzheimer's
- Did the definition of Alzheimer's disease change?
- How your brain works
- Intermittent fasting
- Lecanemab for Alzheimer's disease
- Male depression: Understanding the issues
- MAOIs and diet: Is it necessary to restrict tyramine?
- Marijuana and depression
- Mayo Clinic Minute: 3 tips to reduce your risk of Alzheimer's disease
- Mayo Clinic Minute: Alzheimer's disease risk and lifestyle
- Mayo Clinic Minute: New definition of Alzheimer's changes
- Mayo Clinic Minute: Women and Alzheimer's Disease
- Memory loss: When to seek help
- Monoamine oxidase inhibitors (MAOIs)
- Natural remedies for depression: Are they effective?
- Nervous breakdown: What does it mean?
- New Alzheimers Research
- Pain and depression: Is there a link?
- Phantosmia: What causes olfactory hallucinations?
- Positron emission tomography scan
- Posterior cortical atrophy
- Seeing inside the heart with MRI
- Selective serotonin reuptake inhibitors (SSRIs)
- Serotonin and norepinephrine reuptake inhibitors (SNRIs)
- Treatment-resistant depression
- Tricyclic antidepressants and tetracyclic antidepressants
- Video: Alzheimer's drug shows early promise
- Vitamin B-12 and depression
- Young-onset Alzheimer's
Mayo Clinic does not endorse companies or products. Advertising revenue supports our not-for-profit mission.
- Opportunities
Mayo Clinic Press
Check out these best-sellers and special offers on books and newsletters from Mayo Clinic Press .
- Mayo Clinic on Incontinence - Mayo Clinic Press Mayo Clinic on Incontinence
- The Essential Diabetes Book - Mayo Clinic Press The Essential Diabetes Book
- Mayo Clinic on Hearing and Balance - Mayo Clinic Press Mayo Clinic on Hearing and Balance
- FREE Mayo Clinic Diet Assessment - Mayo Clinic Press FREE Mayo Clinic Diet Assessment
- Mayo Clinic Health Letter - FREE book - Mayo Clinic Press Mayo Clinic Health Letter - FREE book
- Sundowning Late-day confusion
Your gift holds great power – donate today!
Make your tax-deductible gift and be a part of the cutting-edge research and care that's changing medicine.
Nocturnal Awakenings
- First Online: 24 March 2021
Cite this chapter
- Kenneth Lee 4
576 Accesses
Nocturnal awakenings can occur from a wide range of etiologies, including respiratory causes such as sleep disordered breathing, movement disorders such as periodic limb movements, and disruptions secondary to parasomnias. In addition, other etiologies such as nocturnal seizures can cause disruption of sleep and may mimic primary sleep disorders. This can lead to difficulty in diagnosis and optimal treatment. Further complicating this is the fact that nocturnal seizures may result in sleep disruption thus becoming an independent trigger for parasomnia activity at night. In this chapter, we explore two cases of nocturnal awakening and arousal during sleep, which are phenotypically similar but stem from different etiologies.
This is a preview of subscription content, log in via an institution to check access.
Access this chapter
- Available as EPUB and PDF
- Read on any device
- Instant download
- Own it forever
- Compact, lightweight edition
- Dispatched in 3 to 5 business days
- Free shipping worldwide - see info
- Durable hardcover edition
Tax calculation will be finalised at checkout
Purchases are for personal use only
Institutional subscriptions
American Academy of Sleep Medicine. International classification of sleep disorders. 3rd ed. Darien: American Academy of Sleep Medicine; 2014.
Google Scholar
Guilleminault C, Moscovitch A, Leger D. Forensic sleep medicine: nocturnal wandering and violence. Sleep. 1995;18:740–8.
Article CAS Google Scholar
Carskadon MA, Dement WC. Monitoring and staging human sleep. In: Principles and practice of sleep medicine. 5th ed. St. Louis: Elsevier Saunders; 2011. p. 16–26.
Chapter Google Scholar
Attarian H. Treatment options for parasomnias. Neurol Clin. 2010;28:1089–106.
Article Google Scholar
Stallman HM, Kohler M. Prevalence of sleepwalking: a systematic review and meta-analysis. PLoS One. 2016;11(11):e0164769.
Heidbreder A, Frauscher B, Mitterling T. Not only sleepwalking but NREM parasomnia irrespective of the type is associated with HLA DQB1*05:01. J Clin Sleep Med. 2016;12(4):565–70.
Lopez R, Jaussent I, Scholz S, Bayard S, Montplaisir J, Dauvilliers Y. Functional impairment in adult sleepwalkers: a case-control study. Sleep. 2013;36(3):345–51.
PubMed PubMed Central Google Scholar
Parrino L, Grassi A, Milioli G. Cyclic alternating pattern in polysomnography: what it is and what does it mean? Curr Opin Pulm Med. 2014;20(6):533–41.
Guilleminault C, Kirisoglu C, Da Rosa A, Lopes C, Chan A. Sleepwalking, a disorder of NREM sleep instability. Sleep Med. 2006;7(2):163–70.
Tassinari CA, Gardella E, Cantalupo G, et al. Central pattern generators relationships to parasomnias and sleep-related epileptic seizures. Sleep Med Clin. 2012;7:125.
Wong CK, Marshall NS, Grunstein RR, et al. Spontaneous adverse event reports associated with zolpidem in the United States 2003–2012. J Clin Sleep Med. 2017;13(2):223–34.
Ingravallo F, Poli F, Gilmore EV, et al. Sleep-related violence and sexual behavior in sleep: a systematic review of medical-legal case reports. J Clin Sleep Med. 2014;10(8):927–35.
Fois C, Wright MA, Sechi G, Walker MC, Eriksson SH. The utility of polysomnography for the diagnosis of NREM parasomnias: an observational study over 4 years of clinical practice. J Neurol. 2014;262(2):385–93.
Attarian H, Zhu L. Treatment options for disorders of arousal: a case series. Int J Neurosci. 2013;123(9):623–5.
Annegers JF, Hauser WA, Lee JR, Rocca WA. Incidence of acute symptomatic seizures in Rochester, Minnesota, 1935–1984. Epilepsia. 1995;36:327–33.
Tinuper P, et al. From nocturnal frontal lobe epilepsy to sleep-related hypermotor epilepsy: a 35-year diagnostic challenge seizure. Eur J Epilepsy. 2017;44:87–92.
Proserpio P, et al. Epileptic motor behaviors during sleep: anatomo-electro-clinical features. Sleep Med. 2011;12(Suppl 2):S33–8.
Nobili L, Cossu M, Mai R, Tassi L, Cardinale F, Castana L, et al. Sleep-related hyperkinetic seizures of temporal lobe origin. Neurology. 2004;62:482–5.
Herman ST, Walczak TS. Distribution of partial seizures during the sleep–wake cycle Bazil. Neurology. 2001;56(11):1453–9.
Kaleyias J, Arora R, Kothare S. Nocturnal paroxysmal dystonia. In: Kothare S, Ivanenko A, editors. Parasomnias. New York: Springer; 2013.
Scheffer IE, Bhatia KP, Lopes-Cendes I, Fish DR, Marsden CD, Andermann E, et al. Autosomal dominant nocturnal frontal lobe epilepsy. A distinctive clinical disorder. Brain. 1995;118:61–73.
Tinuper P, Cerullo A, Cirignotta F, Cortelli P, Lugaresi E, Montagna P. Nocturnal paroxysmal dystonia with short-lasting attacks: three cases with evidence for an epileptic frontal lobe origin of seizures. Epilepsia. 1990;31:549–56.
Marini C, Guerrini R. The role of the nicotinic acetylcholine receptors in sleep-related epilepsy. Biochem Pharmacol. 2007;74:1308–14.
Bisulli F, Vignatelli L, Provini F, Leta C, Lugaresi E, Tinuper P. Parasomnias and nocturnal frontal lobe epilepsy (NFLE): lights and shadows – controversial points in the differential diagnosis. Sleep Med. 2011;12(Suppl 2):S27–32.
Gibbs SA, Proserpio P, Terzaghi M, Pigorini A, Sarasso S, Russo GL, et al. Sleep-related epileptic behaviors and non-REM-related parasomnias: insights from stereo-EEG. Sleep Med Rev. 2016;25:4–20.
Kothare SV, Kaleyias J. Sleep and epilepsy in children and adolescents. Sleep Med. 2010;11(7):674–68.
Huang YZ, Chu NS. Episodic nocturnal wandering and complex visual hallucination. A case with long-term follow-up. Seizure. 1998;7:67–71.
Derry CP, Davey M, Johns M, et al. Distinguishing sleep disorders from seizures: diagnosing bumps in the night. Arch Neurol. 2006;63(5):705–9.
Derry CP, Duncan JS, Berkovic SF. Paroxysmal motor disorders of sleep: the clinical spectrum and differentiation from epilepsy. Epilepsia. 2006;47:1775–91.
Oldani A, Zucconi M, Asselta R, Modugno M, Bonati MT, Dalpra L, Malcovati M, Tenhini ML, Smirne S, Ferini-Strambi L. Autosomal dominant nocturnal frontal lobe epilepsy: a video polysomnographic and genetic appraisal of 40 patients and delineation of the epileptic syndrome. Brain. 1998;121:205–23.
Download references
Author information
Authors and affiliations.
Department of Neurology, University of Chicago Medical Center, Chicago, IL, USA
Kenneth Lee
You can also search for this author in PubMed Google Scholar
Corresponding author
Correspondence to Kenneth Lee .
Editor information
Editors and affiliations.
Division of Pulmonary, Critical Care, Sleep and Allergy, Department of Medicine, University of Illinois at Chicago, Chicago, IL, USA
Ashima S. Sahni
Department of Neurology, University of California, Davis, Sacramento, CA, USA
Ajay Sampat
Department of Neurology, Northwestern University Feinberg, School of Medicine, Chicago, IL, USA
Hrayr Attarian
Rights and permissions
Reprints and permissions
Copyright information
© 2021 The Author(s), under exclusive license to Springer Nature Switzerland AG
About this chapter
Lee, K. (2021). Nocturnal Awakenings. In: Sahni, A.S., Sampat, A., Attarian, H. (eds) Sleep Disorders. Springer, Cham. https://doi.org/10.1007/978-3-030-65302-6_3
Download citation
DOI : https://doi.org/10.1007/978-3-030-65302-6_3
Published : 24 March 2021
Publisher Name : Springer, Cham
Print ISBN : 978-3-030-65301-9
Online ISBN : 978-3-030-65302-6
eBook Packages : Medicine Medicine (R0)
Share this chapter
Anyone you share the following link with will be able to read this content:
Sorry, a shareable link is not currently available for this article.
Provided by the Springer Nature SharedIt content-sharing initiative
- Publish with us
Policies and ethics
- Find a journal
- Track your research
Call our 24 hours, seven days a week helpline at 800.272.3900

- Professionals
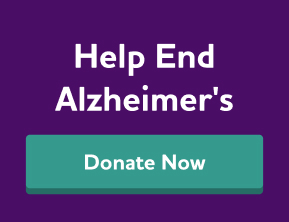
- Younger/Early-Onset Alzheimer's
- Is Alzheimer's Genetic?
- Women and Alzheimer's
- Creutzfeldt-Jakob Disease
- Dementia with Lewy Bodies
- Down Syndrome & Alzheimer's
- Frontotemporal Dementia
- Huntington's Disease
- Mixed Dementia
- Normal Pressure Hydrocephalus
- Posterior Cortical Atrophy
- Parkinson's Disease Dementia
- Vascular Dementia
- Korsakoff Syndrome
- Traumatic Brain Injury (TBI)
- Know the 10 Signs
- Difference Between Alzheimer's & Dementia
- 10 Steps to Approach Memory Concerns in Others
- Medical Tests for Diagnosing Alzheimer's
- Why Get Checked?
- Visiting Your Doctor
- Life After Diagnosis
- Stages of Alzheimer's
- Earlier Diagnosis
- Part the Cloud
- Research Momentum
- Our Commitment to Research
- TrialMatch: Find a Clinical Trial
- What Are Clinical Trials?
- How Clinical Trials Work
- When Clinical Trials End
- Why Participate?
- Talk to Your Doctor
- Clinical Trials: Myths vs. Facts
- Can Alzheimer's Disease Be Prevented?
- Brain Donation
- Navigating Treatment Options
- Lecanemab Approved for Treatment of Early Alzheimer's Disease
- Aducanumab Discontinued as Alzheimer's Treatment
- Medicare Treatment Coverage
- Donanemab for Treatment of Early Alzheimer's Disease — News Pending FDA Review
- Questions for Your Doctor
- Medications for Memory, Cognition and Dementia-Related Behaviors
- Treatments for Behavior
- Treatments for Sleep Changes
- Alternative Treatments
- Facts and Figures
- Assessing Symptoms and Seeking Help
- Now is the Best Time to Talk about Alzheimer's Together
- Get Educated
- Just Diagnosed
- Sharing Your Diagnosis
- Changes in Relationships
- If You Live Alone
- Treatments and Research
- Legal Planning
- Financial Planning
- Building a Care Team
- End-of-Life Planning
- Programs and Support
- Overcoming Stigma
- Younger-Onset Alzheimer's
- Taking Care of Yourself
- Reducing Stress
- Tips for Daily Life
- Helping Family and Friends
- Leaving Your Legacy
- Live Well Online Resources
- Make a Difference
- Daily Care Plan
- Communication and Alzheimer's
- Food and Eating
- Art and Music
- Incontinence
- Dressing and Grooming
- Dental Care
- Working With the Doctor
- Medication Safety
- Accepting the Diagnosis
- Early-Stage Caregiving
- Middle-Stage Caregiving
- Late-Stage Caregiving
- Aggression and Anger
- Anxiety and Agitation
- Hallucinations
- Memory Loss and Confusion
- Sleep Issues and Sundowning
- Suspicions and Delusions
- In-Home Care
- Adult Day Centers
- Long-Term Care
- Respite Care
- Hospice Care
- Choosing Care Providers
- Finding a Memory Care-Certified Nursing Home or Assisted Living Community
- Changing Care Providers
- Working with Care Providers
- Creating Your Care Team
- Long-Distance Caregiving
- Community Resource Finder
- Be a Healthy Caregiver
- Caregiver Stress
- Caregiver Stress Check
- Caregiver Depression
- Changes to Your Relationship
- Grief and Loss as Alzheimer's Progresses
- Home Safety
- Dementia and Driving
- Technology 101
- Preparing for Emergencies
- Managing Money Online Program
- Planning for Care Costs
- Paying for Care
- Health Care Appeals for People with Alzheimer's and Other Dementias
- Social Security Disability
- Medicare Part D Benefits
- Tax Deductions and Credits
- Planning Ahead for Legal Matters
- Legal Documents
- ALZ Talks Virtual Events
- ALZNavigator™
- Veterans and Dementia
- The Knight Family Dementia Care Coordination Initiative
- Online Tools
- Asian Americans and Pacific Islanders and Alzheimer's
- Native Americans and Alzheimer's
- Black Americans and Alzheimer's
- Hispanic Americans and Alzheimer's
- LGBTQ+ Community Resources for Dementia
- Educational Programs and Dementia Care Resources
- Brain Facts
- 50 Activities
- For Parents and Teachers
- Resolving Family Conflicts
- Holiday Gift Guide for Caregivers and People Living with Dementia
- Trajectory Report
- Resource Lists
- Search Databases
- Publications
- Favorite Links
- 10 Healthy Habits for Your Brain
- Stay Physically Active
- Adopt a Healthy Diet
- Stay Mentally and Socially Active
- Online Community
- Support Groups
Find Your Local Chapter
- Any Given Moment
- New IDEAS Study
- Bruce T. Lamb, Ph.D., Chair
- Christopher van Dyck, M.D.
- Cynthia Lemere, Ph.D.
- David Knopman, M.D.
- Lee A. Jennings, M.D. MSHS
- Karen Bell, M.D.
- Lea Grinberg, M.D., Ph.D.
- Malú Tansey, Ph.D.
- Mary Sano, Ph.D.
- Oscar Lopez, M.D.
- Suzanne Craft, Ph.D.
- RFI Amyloid PET Depletion Following Treatment
- About Our Grants
- Andrew Kiselica, Ph.D., ABPP-CN
- Arjun Masurkar, M.D., Ph.D.
- Benjamin Combs, Ph.D.
- Charles DeCarli, M.D.
- Damian Holsinger, Ph.D.
- David Soleimani-Meigooni, Ph.D.
- Donna M. Wilcock, Ph.D.
- Elizabeth Head, M.A, Ph.D.
- Fan Fan, M.D.
- Fayron Epps, Ph.D., R.N.
- Ganesh Babulal, Ph.D., OTD
- Hui Zheng, Ph.D.
- Jason D. Flatt, Ph.D., MPH
- Jennifer Manly, Ph.D.
- Joanna Jankowsky, Ph.D.
- Luis Medina, Ph.D.
- Marcello D’Amelio, Ph.D.
- Marcia N. Gordon, Ph.D.
- Margaret Pericak-Vance, Ph.D.
- María Llorens-Martín, Ph.D.
- Nancy Hodgson, Ph.D.
- Shana D. Stites, Psy.D., M.A., M.S.
- Walter Swardfager, Ph.D.
- ALZ WW-FNFP Grant
- Capacity Building in International Dementia Research Program
- ISTAART IGPCC
- Alzheimer’s Disease Strategic Fund: Endolysosomal Activity in Alzheimer’s (E2A) Grant Program
- Imaging Research in Alzheimer’s and Other Neurodegenerative Diseases
- Zenith Fellow Awards
- National Academy of Neuropsychology & Alzheimer’s Association Funding Opportunity
- Part the Cloud-Gates Partnership Grant Program: Bioenergetics and Inflammation
- Pilot Awards for Global Brain Health Leaders (Invitation Only)
- Robert W. Katzman, M.D., Clinical Research Training Scholarship
- Funded Studies
- How to Apply
- Portfolio Summaries
- Supporting Research in Health Disparities, Policy and Ethics in Alzheimer’s Disease and Dementia Research (HPE-ADRD)
- Diagnostic Criteria & Guidelines
- Annual Conference: AAIC
- Professional Society: ISTAART
- Alzheimer's & Dementia
- Alzheimer's & Dementia: DADM
- Alzheimer's & Dementia: TRCI
- International Network to Study SARS-CoV-2 Impact on Behavior and Cognition
- Alzheimer’s Association Business Consortium (AABC)
- Global Biomarker Standardization Consortium (GBSC)
- Global Alzheimer’s Association Interactive Network
- International Alzheimer's Disease Research Portfolio
- Alzheimer’s Disease Neuroimaging Initiative Private Partner Scientific Board (ADNI-PPSB)
- Research Roundtable
- About WW-ADNI
- North American ADNI
- European ADNI
- Australia ADNI
- Taiwan ADNI
- Argentina ADNI
- WW-ADNI Meetings
- Submit Study
- RFI Inclusive Language Guide
- Scientific Conferences
- AUC for Amyloid and Tau PET Imaging
- Make a Donation
- Walk to End Alzheimer's
- The Longest Day
- RivALZ to End ALZ
- Ride to End ALZ
- Tribute Pages
- Gift Options to Meet Your Goals
- Founders Society
- Fred Bernhardt
- Anjanette Kichline
- Lori A. Jacobson
- Pam and Bill Russell
- Gina Adelman
- Franz and Christa Boetsch
- Adrienne Edelstein
- For Professional Advisors
- Free Planning Guides
- Contact the Planned Giving Staff
- Workplace Giving
- Do Good to End ALZ
- Donate a Vehicle
- Donate Stock
- Donate Cryptocurrency
- Donate Gold & Sterling Silver
- Donor-Advised Funds
- Use of Funds
- Giving Societies
- Why We Advocate
- Ambassador Program
- About the Alzheimer’s Impact Movement
- Research Funding
- Improving Care
- Support for People Living With Dementia
- Public Policy Victories
- Planned Giving
- Community Educator
- Community Representative
- Support Group Facilitator or Mentor
- Faith Outreach Representative
- Early Stage Social Engagement Leaders
- Data Entry Volunteer
- Tech Support Volunteer
- Other Local Opportunities
- Visit the Program Volunteer Community to Learn More
- Become a Corporate Partner
- A Family Affair
- A Message from Elizabeth
- The Belin Family
- The Eliashar Family
- The Fremont Family
- The Freund Family
- Jeff and Randi Gillman
- Harold Matzner
- The Mendelson Family
- Patty and Arthur Newman
- The Ozer Family
- Salon Series
- No Shave November
- Other Philanthropic Activities
- Still Alice
- The Judy Fund E-blast Archive
- The Judy Fund in the News
- The Judy Fund Newsletter Archives
- Sigma Kappa Foundation
- Alpha Delta Kappa
- Parrot Heads in Paradise
- Tau Kappa Epsilon (TKE)
- Sigma Alpha Mu
- Alois Society Member Levels and Benefits
- Alois Society Member Resources
- Zenith Society
- Founder's Society
- Joel Berman
- JR and Emily Paterakis
- Legal Industry Leadership Council
- Accounting Industry Leadership Council
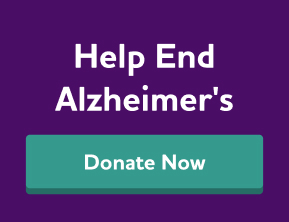
Find Local Resources
Let us connect you to professionals and support options near you. Please select an option below:
Use Current Location Use Map Selector
Search Alzheimer’s Association
- Who's at risk?
Reduce the risk of wandering
Take action when wandering occurs, prepare your home, who's at risk for wandering.
- Returning from a regular walk or drive later than usual.
- Forgetting how to get to familiar places.
- Talking about fulfilling former obligations, such as going to work
- Trying or wanting to “go home” even when at home.
- Becoming restless, pacing or making repetitive movements.
- Having difficulty locating familiar places, such as the bathroom, bedroom or dining room.
- Asking the whereabouts of past friends and family.
- Acting as if doing a hobby or chore, but nothing gets done.
- Appearing lost in a new or changed environment.
- Becoming nervous or anxious in crowded areas, such as markets or restaurants.
- Provide opportunities for the person to engage in structured, meaningful activities throughout the day
- Identify the time of day the person is most likely to wander (for those who experience “ sundowning ,” this may be starting in the early evening.) Plan things to do during this time — activities and exercise may help reduce anxiety, agitation and restlessness.
- Ensure all basic needs are met, including toileting, nutrition and hydration. Consider reducing – but not eliminating – liquids up to two hours before bedtime so the person doesn’t have to use and find the bathroom during the night.
- Involve the person in daily activities, such as folding laundry or preparing dinner. Learn about creating a daily plan .
- Reassure the person if he or she feels lost, abandoned or disoriented.
- If the person is still safely able to drive, consider using a GPS device to help if they get lost.
- If the person is no longer driving, remove access to car keys — a person living with dementia may not just wander by foot. The person may forget that he or she can no longer drive.
- Avoid busy places that are confusing and can cause disorientation, such as shopping malls.
- Assess the person’s response to new surroundings. Do not leave someone with dementia unsupervised if new surroundings may cause confusion, disorientation or agitation.
- Decide on a set time each day to check in with each other.
- Review scheduled activities and appointments for the day together.
- If the care partner is not available, identify a companion for the person living with dementia as needed.
- Consider alternative transportation options if getting lost or driving safely becomes a concern.
As the disease progresses and the risk for wandering increases, assess your individual situation to see which of the safety measures below may work best to help prevent wandering.
Home Safety Checklist
Download, print and keep the checklist handy to prevent dangerous situations and help maximize the person living with dementia’s independence for as long as possible.
- Place deadbolts out of the line of sight, either high or low, on exterior doors. (Do not leave a person living with dementia unsupervised in new or changed surroundings, and never lock a person in at home.)
- Use night lights throughout the home.
- Cover door knobs with cloth the same color as the door or use safety covers.
- Camouflage doors by painting them the same color as the walls or covering them with removable curtains or screens.
- Use black tape or paint to create a two-foot black threshold in front of the door. It may act as a visual stop barrier.
- Install warning bells above doors or use a monitoring device that signals when a door is opened.
- Place a pressure-sensitive mat in front of the door or at the person's bedside to alert you to movement.
- Put hedges or a fence around the patio, yard or other outside common areas.
- Use safety gates or brightly colored netting to prevent access to stairs or the outdoors.
- Monitor noise levels to help reduce excessive stimulation.
- Create indoor and outdoor common areas that can be safely explored.
- Label all doors with signs or symbols to explain the purpose of each room.
- Store items that may trigger a person’s instinct to leave, such as coats, hats, pocketbooks, keys and wallets.
- Do not leave the person alone in a car.
- Consider enrolling the person living with dementia in a wandering response service.
- Ask neighbors, friends and family to call if they see the person wandering, lost or dressed inappropriately.
- Keep a recent, close-up photo of the person on hand to give to police, should the need arise.
- Know the person’s neighborhood. Identify potentially dangerous areas near the home, such as bodies of water, open stairwells, dense foliage, tunnels, bus stops and roads with heavy traffic.
- Create a list of places the person might wander to, such as past jobs, former homes, places of worship or a favorite restaurant.
When someone with dementia is missing
Begin search-and-rescue efforts immediately. Many individuals who wander are found within 1.5 miles of where they disappeared.
- Start search efforts immediately. When looking, consider whether the individual is right- or left-handed — wandering patterns generally follow the direction of the dominant hand.
- Begin by looking in the surrounding vicinity — many individuals who wander are found within 1.5 miles of where they disappeared.
- Check local landscapes, such as ponds, tree lines or fence lines — many individuals are found within brush or brier.
- If applicable, search areas the person has wandered to in the past.
- If the person is not found within 15 minutes, call 911 to file a missing person’s report. Inform the authorities that the person has dementia.
Other pages in Stages and Behaviors
- Care Options
- Caregiver Health
- Financial and Legal Planning for Caregivers
Related Pages
Connect with our free, online caregiver community..
Join ALZConnected
Our blog is a place to continue the conversation about Alzheimer's.
Read the Blog
The Alzheimer’s Association is in your community.
Keep up with alzheimer’s news and events.
Prevalence and comorbidity of nocturnal wandering in the U.S. adult general population
Affiliation.
- 1 Stanford Sleep Epidemiology Research Center, School of Medicine, Stanford University, Stanford, CA, USA. [email protected]
- PMID: 22585435
- PMCID: PMC3467644
- DOI: 10.1212/WNL.0b013e3182563be5
Objective: To assess the prevalence and comorbid conditions of nocturnal wandering with abnormal state of consciousness (NW) in the American general population.
Methods: Cross-sectional study conducted with a representative sample of 19,136 noninstitutionalized individuals of the U.S. general population ≥18 years old. The Sleep-EVAL expert system administered questions on life and sleeping habits; health; and sleep, mental, and organic disorders (DSM-IV-TR; International Classification of Sleep Disorders, version 2; International Classification of Diseases-10).
Results: Lifetime prevalence of NW was 29.2% (95% confidence interval [CI] 28.5%-29.9%). In the previous year, NW was reported by 3.6% (3.3%-3.9%) of the sample: 1% had 2 or more episodes per month and 2.6% had between 1 and 12 episodes in the previous year. Family history of NW was reported by 30.5% of NW participants. Individuals with obstructive sleep apnea syndrome (odds ratio [OR] 3.9), circadian rhythm sleep disorder (OR 3.4), insomnia disorder (OR 2.1), alcohol abuse/dependence (OR 3.5), major depressive disorder (MDD) (OR 3.5), obsessive-compulsive disorder (OCD) (OR 3.9), or using over-the-counter sleeping pills (OR 2.5) or selective serotonin reuptake inhibitor (SSRI) antidepressants (OR 3.0) were at higher risk of frequent NW episodes (≥2 times/month).
Conclusions: With a rate of 29.2%, lifetime prevalence of NW is high. SSRIs were associated with an increased risk of NW. However, these medications appear to precipitate events in individuals with a prior history of NW. Furthermore, MDD and OCD were associated with significantly greater risk of NW, and this was not due to the use of psychotropic medication. These psychiatric associations imply an increased risk due to sleep disturbance.
Publication types
- Research Support, N.I.H., Extramural
- Research Support, Non-U.S. Gov't
- Age Factors
- Aged, 80 and over
- Community Health Planning
- Comorbidity
- Confidence Intervals
- Cross-Sectional Studies
- Depressive Disorder / epidemiology
- Logistic Models
- Mental Disorders / epidemiology
- Middle Aged
- Risk Factors
- Sex Factors
- Somnambulism / diagnosis
- Somnambulism / epidemiology*
- Substance-Related Disorders / epidemiology
- Surveys and Questionnaires
- United States / epidemiology
- Young Adult
Grants and funding
- R01NS044199/NS/NINDS NIH HHS/United States
ORIGINAL RESEARCH article
Commonalities and differences in nrem parasomnias and sleep-related epilepsy: is there a continuum between the two conditions.
- 1 Department of Medicine and Surgery, Sleep Disorders Center, University of Parma, Parma, Italy
- 2 Unit of Neuroscience & Interdepartmental Center of Robust Statistics, Department of Medicine and Surgery, University of Parma, Parma, Italy
Introduction: Differential diagnosis between disorders of arousal (DoA) and sleep-related hypermotor epilepsy (SHE) often represents a clinical challenge. The two conditions may be indistinguishable from a semiological point of view and the scalp video-polysomnography is often uninformative. Both disorders are associated with variable hypermotor manifestations ranging from major events to fragments of a hierarchical continuum of increasing intensity, complexity, and duration. Given their semiological overlap we decided to explore the sleep texture of DoA and SHE seeking for similarities and differences.
Methods: We analyzed sleep macrostructure and CAP (cyclic alternating pattern) parameters in a cohort of 35 adult DoA patients, 40 SHE patients and 24 healthy sleepers, all recorded and scored in the same sleep laboratory. Nocturnal behavioral manifestations included minor motor events, paroxysmal arousals and major attacks in SHE, and simple, rising, or complex arousal movements in DoA.
Results: Compared to healthy controls, DoA and SHE showed similar amounts of sleep efficiency, light sleep, deep sleep, REM sleep, CAP subtypes. Both groups also showed slow wave sleep fragmentation and an increased representation of stage N3 in the second part of the night. The only discriminating elements between the two conditions regarded sleep length (more reduced in DoA) and sleep instability (more elevated in SHE). In DoA recordings, all motor episodes arose from NREM sleep: 37% during light NREM stages and 63% during stage N3 (simple arousal movements: 94%). In SHE recordings, 57% of major attacks occurred during stage N3.
Conclusions: So far, emphasis has been placed on the differentiation of sleep-related epilepsy and NREM arousal disorders. However, the impressive analogies between DoA and SHE suggest the existence of an underestimated continuum across the conditions, linked by increased levels of sleep instability, higher amounts of slow wave sleep and NREM/REM sleep imbalance. Sleep texture is extremely similar in the two conditions, although CAP metrics disclose quantitative differences. In particular, SHE patients show a higher arousal instability compared to DoA subjects. Given their clinical and epidemiological overlap, a common genetic background is also hypothesized. In such a perspective, we suggest that the consolidated dichotomy DoA vs. SHE should be reappraised.
Introduction
Disorders of arousal (DoA) encompass heterogeneous motor behaviors (parasomnias) during non-REM sleep, e.g., sleepwalking, sleep terror, and confusional arousals, arising as a result of incomplete awakening ( 1 ).
DoA can be triggered by sleep deprivation, sleep disorders, medication, or psychosocial stressors and are accompanied by variable degrees of autonomic activation. Misperception, mental confusion, partial unresponsiveness to external stimuli, and retrograde amnesia are commonly reported during DoA episodes ( 2 ). Given its association with complex hypermotor behaviors and abnormal arousal reactions during sleep, the differential diagnosis between DoA and sleep-related hypermotor epilepsy (SHE) is often a challenging issue for clinicians. Both sleep disorders can either persist from childhood or appear de novo and may require different treatment strategies. DoA include a spectrum of disorders encompassing different motor manifestations with increasing semiological complexity which have been classified ( 3 ) as simple arousal movements (SAM), rising arousal movements (RAM) and complex arousal movements (CAM) and may occur in the same patient and in the same night. SHE itself is part of a spectrum ranging from minor motor events (MME), paroxysmal arousals (PA) and major attacks ( 4 – 8 ), often coexisting in the same patient and sometimes in the same night.
The clinical and polysomnographic differentiation between SHE, parasomnias and physiologic movements during sleep has always raised semiological difficulties and according to some authors a clearcut distinction cannot be carried out without video-polysomnographic analysis (v-PSG) ( 9 ). In contrast, Vignatelli et al. ( 10 ) describe major disagreement among sleep experts and trainees in distinguishing between PA and non-epileptic arousals. Other studies underlined the discriminatory informations provided by the sleep stage at onset, while ictal EEG features appear less useful ( 11 ).
DoA are traditionally considered as manifestations of fragmentary arousals occurring mostly during slow-wave sleep (SWS) ( 12 ): the mind is asleep, but the motor system is awake. Rhythmic movements within DoA are generally rare and may be seen either as part of exploratory movements in abortive sleepwalking or as part of complex DoA. In contrast, behaviors in SHE often display rhythmic components, including body rocking and rolling, bipedal cycling, and kicking. The differential diagnosis between DoA and SHE also relies on frequency and distribution pattern of the events: DoA episodes occur sporadically and less stereotyped in the first part of the night (dominated by deep NREM sleep) and are boosted by stage changes; SHE events are more frequent and highly stereotyped, prevail during light NREM stages, and may be preceded by abrupt arousal ( 8 ).
As behavioral events peculiar to DoA and SHE may occur in the same patient during the same night, differential diagnosis may become complicated, especially when only minor/mild events are available in the v-PSG recording. While major attacks in SHE are typically stereotyped and triggered by paroxysmal discharges, minor motor events may widely vary and appear similar to physiological movements during sleep. Furthermore, the association between minor motor events and epileptiform discharges is weak: it has been suggested that the former could reflect the activation of some innate motor patterns, not necessarily linked to paroxysmal events ( 7 ). In DoA patients, the awake EEG is normal, while PSG recordings often document the abrupt appearance of high-amplitude rhythmic slow waves before the episodes, followed by the persistence of either partial or complete sleep activity in the post-arousal recordings ( 1 ).
Brain activity during sleep is physiologically controlled by two driving forces: the sleep-promoting system and the arousal-promoting system, the latter connecting the sleeper with the surrounding world and crucial to restore wakefulness ( 13 ). This interplay is mirrored by the dynamic texture of sleep, which, within certain ranges, warrants flexible and adaptive strategies. Within NREM sleep stages, phasic EEG events are lumped in periodic clusters which define a cyclic alternating pattern (CAP). Recognized as the EEG biomarker of sleep instability, CAP is composed of a phase A of greater arousal (k-complexes, delta burst, polyphasic bursts, arousals) and a phase B of lesser arousal (baseline interval between consecutive A phases). While sleep stages and cycles are the expression of sleep macrostructure, CAP oscillations organized in sequences constitute sleep microstructure, which occurs either spontaneously or evoked by external stimuli and yields to consistent autonomic reactions ( 14 ). As the A phases of CAP encompass different transient events, they are classified as subtypes A1, A2, and A3, based on reciprocal proportion of high-voltage slow waves (EEG synchrony) and low-amplitude fast rhythms (EEG desynchrony). In the physiological architecture of sleep, subtypes A1 parallel the homeostatic process, while subtypes A2 and A3 are closely linked to the ultradian cyclicity. During the descending slope of sleep cycles CAP sequences preserve sleep against perturbations, boosting SWS, whereas during the ascending slope of the sleep cycle, microstructural fluctuations lighten sleep, and prepare the onset of REM periods. Therefore, CAP sequences can provoke both “arousal promoting” and “sleep promoting” reactions, depending on the background homeostatic pressure, and on the ongoing sleep stages ( 15 , 16 ).
Previous studies showed a significant increase of CAP both in SHE ( 14 ) and in DoA patients ( 17 ) compared to healthy controls. Moreover, clinical events in both SHE and DoA are often triggered by an arousal event (phase A of CAP) indicating that SHE and DoA share common sleep features which can explain why it can become extremely difficult to distinguish the two conditions and why the same patient can present both seizures and parasomnias.
So far, particular emphasis has been placed on the differentiation of SHE and NREM arousal disorders ( 18 ). However, the two conditions share an impressive amount of common features. In particular, periodism, the attacks coinciding with the typical CAP recurrence ( 19 ), modulates both nocturnal epileptic ( 20 ) and parasomnic episodes ( 17 , 21 ). In such a perspective, we suggest that the consolidated dichotomy DoA vs. SHE should be reappraised. To explore boundaries, gaps and overlaps in the two conditions, standard PSG measures, CAP parameters, and video findings of adult subjects with DoA were analyzed and compared with the data of age-balanced SHE patients and healthy controls recorded and scored in the same sleep laboratory.
Materials and Methods
We reviewed the database of the Sleep Disorders Center at Parma University Hospital selecting patients with diagnosis of DoA who underwent nocturnal video-PSG in the time period between 2007 and 2019. A total of 234 patients were scrutinized, 199 patients were excluded for variable reasons including: coexistence of sleep disorders others than DoA, concomitant psychiatric or neurological conditions, incomplete follow-up or unavailability of video-PSG recording. We included all consecutive adult patients (≥ 18 years old) with at least 2 neurological visits and an overnight lab-setted v-PSG who received a diagnosis of DoA according to the ICSD criteria (International classification of Sleep Disorders, III Edition, American Academy of Sleep Medicine, 2014). A randomly selected group of patients with a clinical history of paroxysmal arousal, nocturnal wandering or hyperkinetic seizures, composed the SHE group ( 14 ), based on the diagnostic criteria established in 2016 ( 8 ). Eligible healthy controls were paid volunteers free of psychiatric, neurologic, and/or medical disorders, recruited through advertisement at the university hospital.
Exclusion criteria for all the subjects (patients and controls) were the following: (1) concomitant neurological, psychiatric or any other sleep disorders; (2) intake of medications known to influence sleep.
For each DoA patient we collected complete demographical and clinical data from medical recording and then anonymously abstracted them using a standardized data extraction spreadsheet. Specifically we recorded informations relative to age at onset, episodes frequency (divided in low: 1–2 per month; moderate: 1 per week; high: > 1 per week), previous personal medical history, family history for sleep disorders, with specific attention to NREM-parasomnia. Daytime sleepiness was assessed by means of the Epworth Sleepiness Scale (ESS): a score > 10 was considered clinically relevant. All enrolled patients carried out at least one lab-set full-night v-PSG recording.
The clinical, demographic and v-PSG features regarding SHE patients and healthy sleepers were collected from the Sleep Disorders Center at Parma University Hospital database. The major findings of epileptic patients and normal controls were published in a previous report ( 14 ). The study was regularly approved by the Local Ethics Committee with protocol number 9/2019/OSS * /AOUPR.
PSG Evaluation
PSG recording was based on the international 10:20 system with 19 EEG channels on the scalp (Fp1, Fp2, F3, F4, F7, F8, C3, C4, P3, P4, O1, O2, T3, T4, T5, T6, FZ, CZ, PZ) referenced on mastoid, EOG for both eyes, EMG of the mentalis and limb muscles, ECG and synchronized audio-visual recording. A standard calibration of 50 mV/ mm with a time constant of 0.1 s and a high frequency filter in the 30 Hz range were applied. For all subjects bedtime was fixed at 10.30 pm. A resting wakefulness EEG of at least 20 min of duration was evaluated by a neurologist with expertise in epilepsy and sleep disorders. The detection of an apnea–hypopnea index >5/h and/or periodic limb movement (PLM) index >15/h of sleep represented exclusion criteria. Specifically “apneas” were defined as complete cessation of airflow for > 10 s, “hypopneas” were characterized by at least 30% drop in airflow from baseline value for at least 10 s and accompanied by either 3% reduction in SatO2% with respect to pre-event baseline and/or by an EEG arousal; “respiratory effort related arousal” were defined as sequences of breaths lasting at least 10 s and associated with increased respiratory efforts leading to arousal from sleep, not fulfilling diagnostic criteria for apnea nor hypopnea, in line with American Academy of Sleep Medicine (AASM) scoring rules ( 22 ).
Sleep Macrostructure
PSG scoring was carried out according to AASM rules ( 22 ). We measured sleep efficiency (SE), total sleep time (TST), stage 1 (N1) sleep time, stage 2 (N2) sleep time, stage 3 (N3) sleep time, REM sleep time.
Sleep Microstructure
CAP was performed following standardized guidelines ( 23 ) using Embla REM-logic software. For CAP analysis the following variables were evaluated: CAP rate (CAP time/total non-REM time × 100), CAP rate in NREM stages; CAP subtypes A1, subtypes A2, and subtypes A3.
Classification of Nocturnal Motor Episodes
When DoA patients presented motor episodes during the recording night we described the clinical semiology and the sleep stage distribution. According to Loddo et al. ( 3 ), behavioral patterns of DoA patients were classified as: simple arousal movements (SAMs), rising arousal movements (RAMs) and complex arousal movements (CAMs). More specifically, SAMs referred to simple head movements comprehensive of head flexion/extension (SAM-A), with or without limb (SAM-B), or trunk minor motor activation (SAM-C). SAMs are far the commonest nocturnal motor manifestation in adults with DoA. RAMs are characterized by more complex in-bed motor patterns (trunk flexion or sitting) sometimes associated with speaking. Finally CAMs represent the most elaborate DoA motor pattern: patients with CAMs may leave their bed, manipulate objects, scream, or walk in the room.
Nocturnal epileptic seizures were classified as minor motor events, paroxysmal arousals and major events. Specifically minor motor events were represented by brief, simple and stereotyped movements involving either head, trunk or limbs; paroxysmal arousals were associated with sudden arousal and stereotyped motor activation with variable association of autonomic reactions and/or vocalization, lasting from 5 to 10 s and finally major events were the most complex episodes, consisting in complex stereotyped hypermotor patterns (including tonic–dystonic or hyperkinetic seizures and epileptic nocturnal wandering) with frequent autonomic activation, lasting on average 20–30 s ( 6 ). Only epileptic motor events supported by v-PSG evidence were taken into consideration.
Statistical Analysis
Statistical analysis was performed using the open-source software Jamovi ( 24 ). All quantitative data were expressed as mean and standard deviation (SD). Qualitative data were reported as absolute frequency and percentage. A one-way ANOVA test assessed the differences among the mean values in the 3 groups (healthy controls, DoA, SHE). Normality of the data and homogeneity of variances were tested by the Shapiro-Wilk test and Levene's test, respectively. Categorical data were analyzed by the Pearson's chi-square test. Statistical significance was set at p < 0.05. Tukey post-hoc test was used to explore differences between groups after ANOVA. Effect size were also reported for ANOVA (Cohen's f and partial eta-squared) ( 25 ) and for Chi-square (Cramer's V) to measure the strength of the relationship between variables.
Due to strict inclusion criteria the final sample included 35 DoA subjects (12 female and 23 male, with a mean age of 28 ± 5 years). The SHE group included 40 subjects (20 male and 20 female; mean age: 31 ± 10 years). The control population encompassed 24 subjects (12 male and 12 female; mean age: 28 ± 7 years). The three groups showed similar age distribution ( Table 1 ).
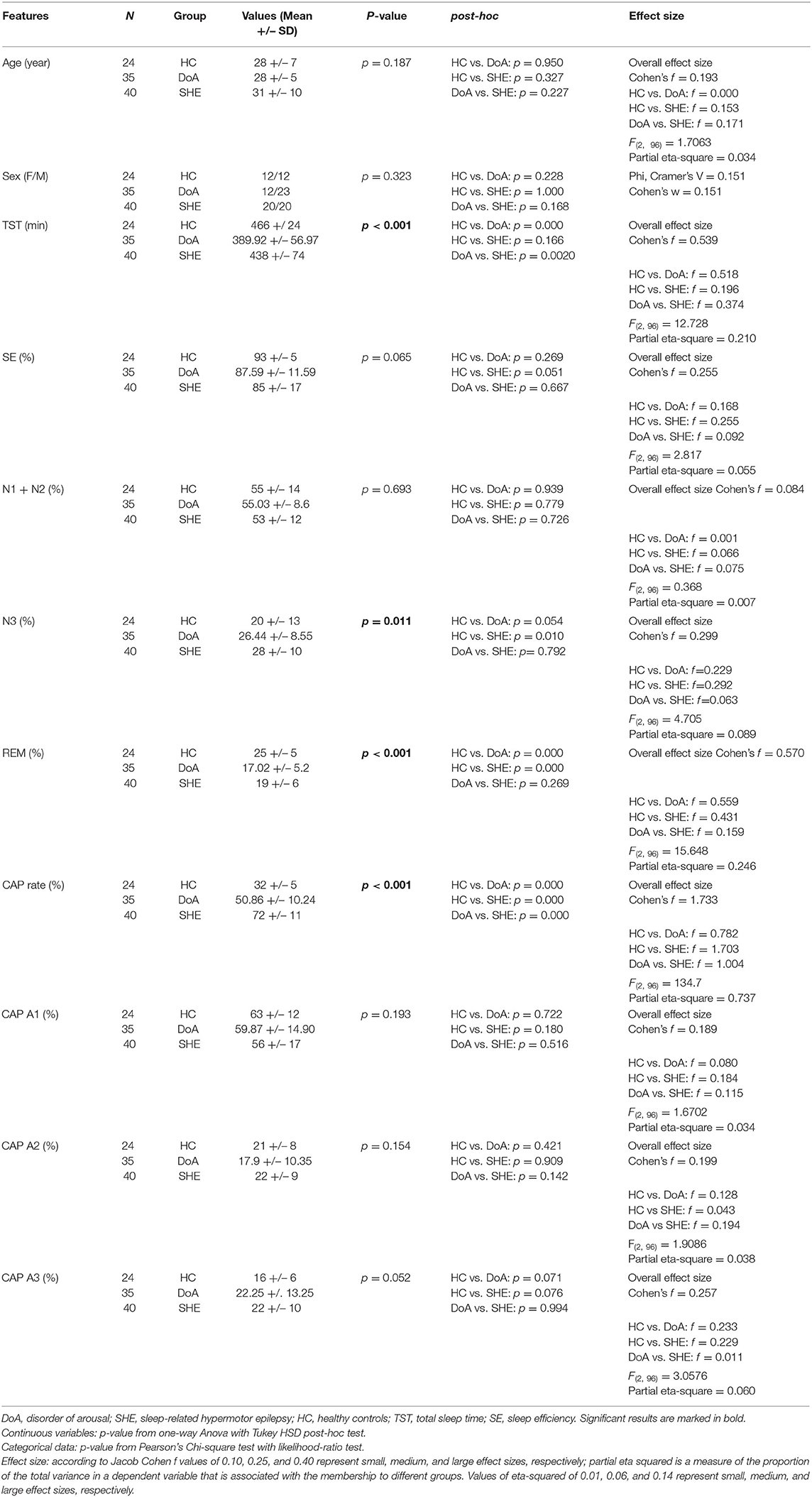
Table 1 . Sleep macro and microstructure features in the three groups.
A family history for sleep disorders was documented in 6 cases (17%), disease onset during childhood was reported by 22 patients (63%), while the other 13 subjects (37%) developed NREM sleep parasomnia after the age of 18. Five patients (14%) presented high frequency of DoA episodes (>1 per week), 15 patients (43%) reported usually one episode per week and the remaining 15 patients (43%) had a mean of one or two episodes per month. Five patients (14%) suffered from concomitant psychiatric diseases including depression, anxiety and panic attacks. Daytime sleepiness (measured by ESS, cut-off >10) was found in 9 patients (23%).
In most patients with SHE, nocturnal motor events were already present at childhood. One patient suffered from mild perinatal hypoxia, two presented febrile convulsion during childhood and one had minor head trauma. No patient reported a family history of epilepsy, but 10 patients described parasomnias in first-degree relatives. Diurnal seizures were never reported and no patient showed coexisting neurologic, psychiatric or medical disorders known to affect sleep architecture. All SHE patients complained of excessive daytime sleepiness (ESS: 16 ± 4). Wakefulness EEG showed focal epileptiform abnormalities only in a minority of cases (5/40, 12.5%). Semiology of nocturnal episodes included paroxysmal arousals, focal tonic-dystonic seizure, hyperkinetic seizure, or prolonged motor behavior including epileptic nocturnal wandering. Brain MRI was unremarkable in all SHE patients. According to 2017 ILAE classification ( 26 ) all included subjects were classified as affected by focal epilepsy of unknown etiology.
The standard sleep measures in the three groups are detailed in Table 1 . One-way ANOVA highlighted significant differences between groups with respect to TST, N3 and REM representation. Tukey post-hoc test revealed that, compared to healthy controls, TST was reduced significantly in DoA (−76 min, p = 0.0001) but not significantly in SHE (−28 min, p = 0.166). TST was also significantly lower in DoA compared to SHE (−48 min, p = 0.002). Overall, SE was not modified ( p = 0.065) but both DoA (88%) and SHE (85%) presented values <90%.
The three groups showed similar amounts of light sleep (N1 + N2). Overall stage N3 was significantly different between the three groups ( p = 0.011), being enhanced in DOA (+6%, p = 0.054) and in SHE (+8%, p = 0.010) with respect to healthy sleepers but no difference was found between the two clinical conditions ( p = 0.792). Compared to healthy sleepers, REM sleep was reduced ( p < 001) in DoA (−8%, p = 0.0001) and in SHE (−6%, p = 0.0001) with similar values in both conditions ( p = 0.269).
In the 9 DoA patients complaining of excessive daytime sleepiness compared to the remaining 26 DoA subjects with an ESS ≤ 10, higher percentages of N3 (33 ± 9 vs. 24 ± 7; p = 0.004) and lower amounts of REM sleep (13% ± 5 vs. 18% ± 5; p = 0.014) were found.
Tables 1 , 2 showed the mean microstructural data in the three groups. Overall one-way ANOVA described significant differences between the three groups with respect to CAP rate, CAP rate in N2 and CAP rate in N3.
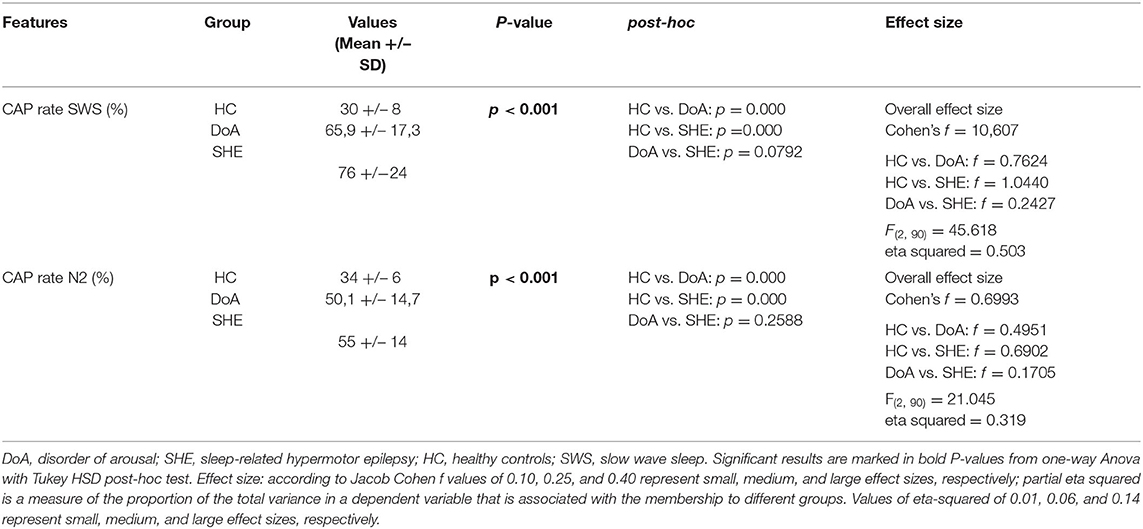
Table 2 . CAP rate during stage N2 and N3 of NREM sleep in the three groups.
In details, compared to healthy controls, CAP rate values were higher ( p <0.001) in DoA (+ 19%) and even more elevated in SHE (+ 40%), with significant differences between the two conditions ( p = 0.0001). In the three groups, CAP subtypes maintained the physiological ranking A1 > A3 > A2, with a similar increased representation of phases A3 ( p = 0.052) in DoA (+6%, p = 0.071) and SHE (+6%, p = 0.076), presenting trends toward significancy.
CAP rate values in stages N2 and N3 were significantly higher in both DoA and SHE compared to healthy sleepers ( p < 0.001) (details in Table 2 ).
EEG Features
Epileptiform discharges during wakefulness occurred only in 5 SHE patients. During sleep, EEG abnormalities were identified in 28 of the 35 DoA patients (80%) and in all the SHE patients, the vast majority being represented by focal spikes. Comparing DoA patients with EEG abnormalities vs. DoA patients showing normal EEG no significant differences were detected with respect to sleep macrostructural and microstructural features: TST ( p = 0,140), SE ( p = 0,367), N1 + N2% ( p = 0,297), N3% ( p = 0,728), REM% ( p = 0,080), CAP rate ( p = 0,911), CAP subtype A1 ( p = 0,677), A2 ( p = 1,000), A3 ( p = 0,676).
During v-PSG registration at least one DoA episode occurred in 27 patients (77%). All episodes were recorded during NREM sleep and in most cases arised from a phase A of CAP ( Figure 1 ): 37 % occurred during light NREM sleep (stages N1 and N2) and 63% during N3. DoA episodes included episodes of confusional arousals, sleep terrors, sleep-walking, vocalizations (moaning or mumbling), laughing, simple movements (face or nose touching), eye opening, oro-masticatory movements. A total of 48 episodes were recorded: they were classified as simple arousal movements (SAM) (45 episodes), rising arousal movements (RAM) (2 episodes) or complex arousal movements (CAM) (1 episode). The SAM group included 9 SAM-A (20%), 23 SAM-B (51%) and 13 SAM-C (29%) patterns.
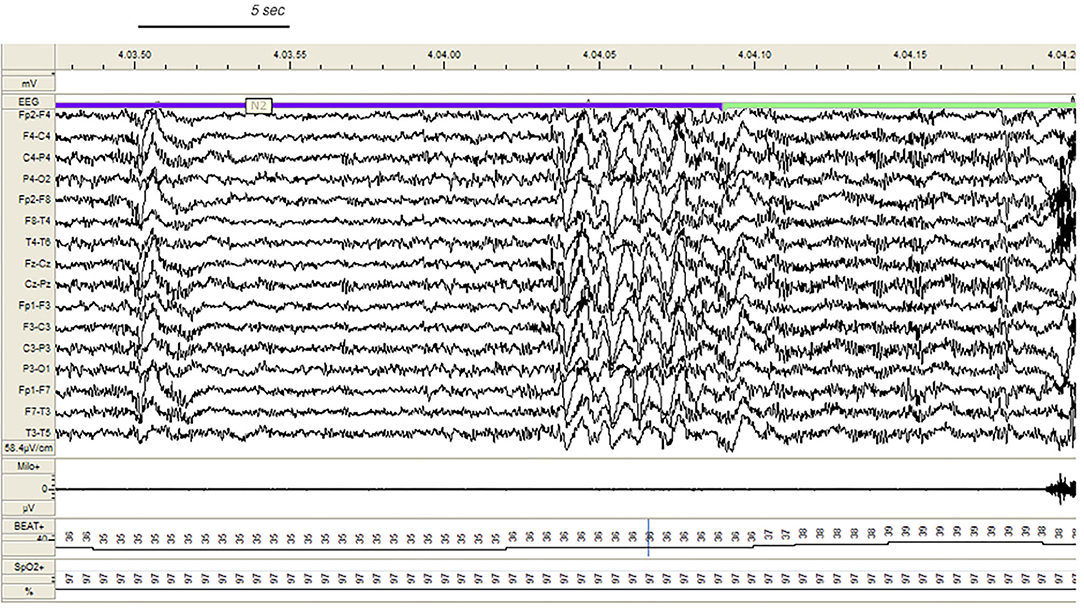
Figure 1 . Confusional arousal beginning during stage N2 in a DoA patient. A high amplitude EEG burst of spiky slow waves precedes the episode. Notice the coexistence of autonomic arousal (heart rate acceleration) and the absence of respiratory events; EEG sensitivity 58.4 mV/cm; Milo+, chin electromyography; BEAT, heart frequency; SpO2+, oxygen saturation.
In the SHE group, 33 patients (83%) reported multiple nocturnal episodes every night, whereas self-reported or witnessed seizures recurred weekly in 7 patients. Approximately 60% of the total amount of NREM sleep seizures arose from N2, but most major events (57%) showed a preferential occurrence during SWS ( Figure 2 ). Ninety percent of total NREM seizures occurred during a CAP sequence, and CAP-related seizures always occurred in association with a phase A.
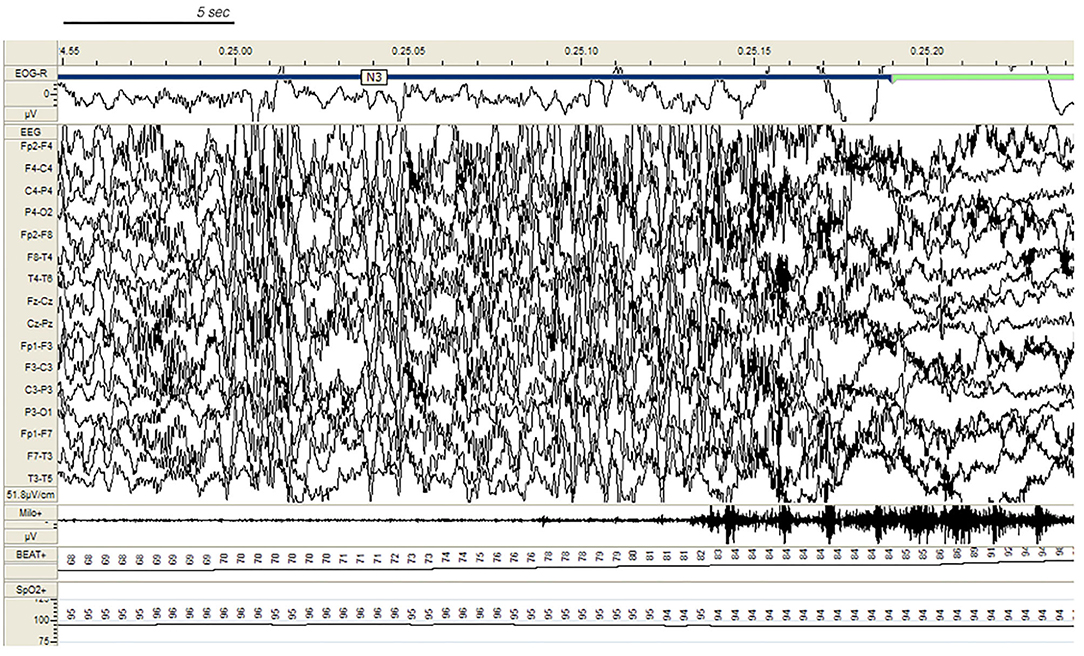
Figure 2 . Paroxysmal arousal in a patient with SHE beginning during stage N3. Bursts of generalized high amplitude epileptiform discharges can be appreciated. Autonomic activation coexists. EEG sensitivity 51.8 mV/cm; Milo+: chin electromyography; BEAT, heart frequency; SpO2+, oxygen saturation.
Both DoA and SHE presented impressive overlaps of sleep macro- and microstructural parameters. Compared to healthy controls, the two sleep disorders showed similar amounts of sleep efficiency, light sleep (N1+N2), deep sleep (N3), REM sleep, CAP subtypes (A1, A2, A3). Both groups also showed SWS fragmentation and an increased representation of stage N3 in the second part of the night. The only discriminating elements between the two conditions regarded sleep length (TST more reduced in DoA) and sleep instability (CAP rate more elevated in SHE).
Sleep Texture
The PSG alterations of DoA and SHE seem to be peculiar to the conditions. Indeed other sleep disorders including insomnia, periodic limb movements, sleep disordered breathing may present reduced sleep efficiency and increased sleep instability, but a significant increase of stage N3 is a really uncommon finding. In our DoA sample, the excess of SWS probably accounted for the lack of sleepiness in most patients (77%). On the contrary, in the SHE group, the protective action of N3 on daytime vigilance was counteracted by an excessive amount of CAP rate, a magnitude already described in severe obstructive sleep apnea ( 27 – 29 ), which is often accompanied by daytime sleepiness. The supplement of unstable sleep in the SHE group is fueled by a number of disturbing factors including ictal and interictal EEG paroxysms acting as noise equivalents on the neural circuitry ( Figure 3 ). In a study on SHE patients ( 30 ) treated with antiepileptic agents, CAP rate dropped from 71% (no medication) to 59% (medication), remaining widely above the expected physiological value of 33% (healthy controls). Effective treatment reduced the total amount of sleep seizures of approximately 25%, but the persistence of EEG discharges fanned arousal oscillations during NREM sleep, producing an increase of reactive A phases and therefore boosting high CAP rate values. It is interesting to notice that medication in SHE patients was associated with an additional growth of stage N3 (+3%), which was impressively high (28%) even without medication.
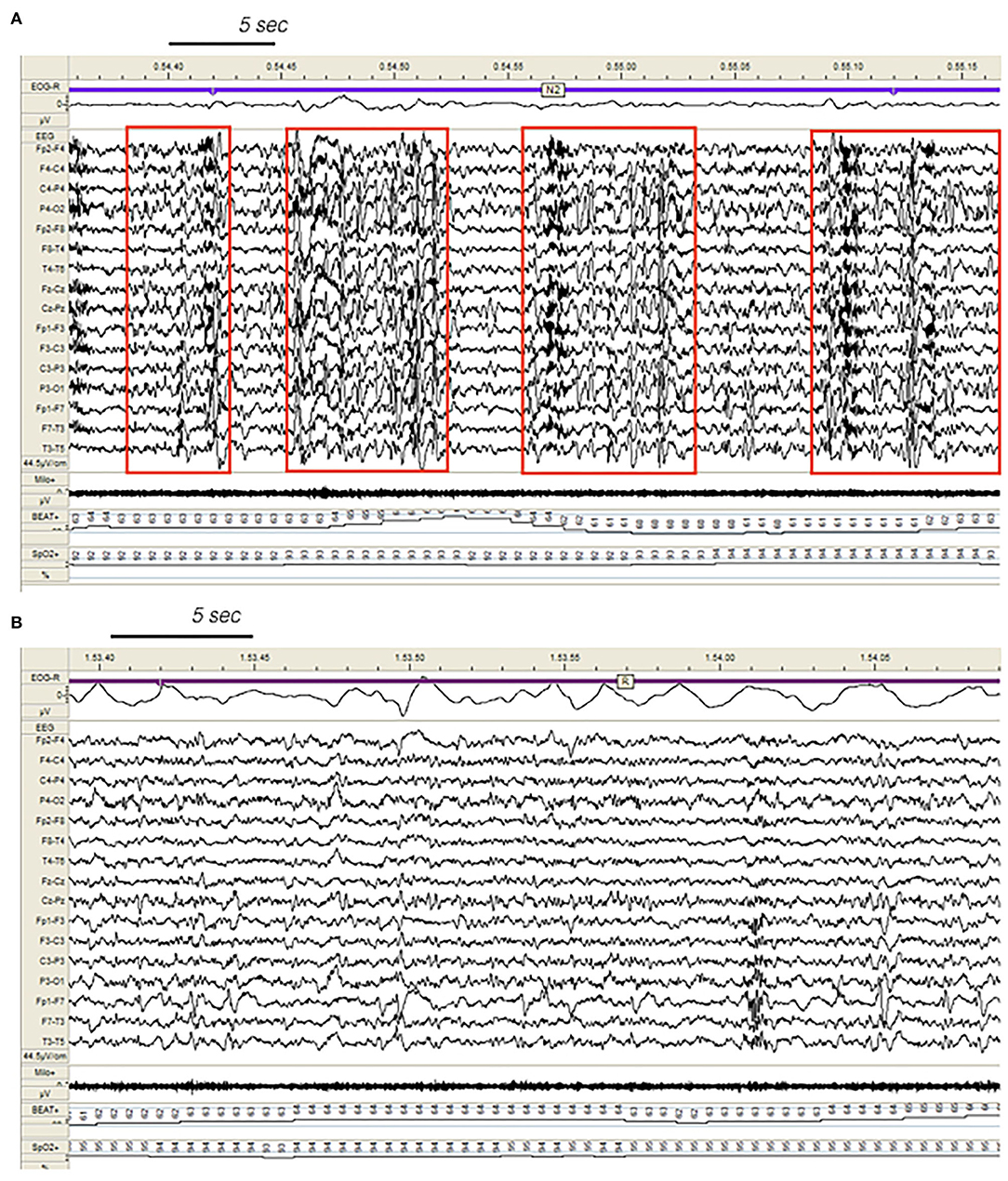
Figure 3 . Dynamics of epileptiform discharges during NREM and REM sleep in a patient with SHE. (A) Bursts of generalized epileptiform discharges enhancing cyclic alternating pattern (CAP) fluctuations during stage N2 in a patient with SHE. Red boxes highlight CAP cycles. Transient heart rate fluctuations consistent with sleep instability. (B) REM sleep constrains epileptic phenomena and may provide information on the localization of seizure onset zone (left focal fronto-temporal spikes and sharp-waves). EEG sensitivity 44.5 mV/cm; Milo+, chin electromyography; BEAT, heart frequency; SpO2+, oxygen saturation.
Trait vs. State Features
DoA and SHE share a number of specific EEG trait-markers and state-markers embedded within their sleep texture. Whether the elevated percentages of N3 are an intrinsic feature (trait) of both SHE and DoA or the compensatory by-product of a non-consolidated SWS due to motor events and/or EEG paroxysms occurring in NREM sleep (state) remains an open question. Probably, both assumptions stem from a common root. In DoA patients, impairment of sleep intensity and depth ( 31 , 32 ) determines vulnerable and discontinuous slow waves during stage N3 ( 33 ). DoA sleep recordings are also characterized by an excessive fragmentation of SWS independent of concomitant parasomniac behaviors ( 34 ). Moreover, patients with DoA suffer from more frequent and longer arousals and awakenings from N3 than controls ( 35 ). Factors interfering with the build-up and maintenance of SWS, such as an excess and/or an abnormal distribution of CAP could also play a role in the pathophysiology of the disease. Accordingly, both SHE and DoA recordings were characterized by high amounts of SWS in the second half of the night: probably the result of an adaptive intra-night homeostatic recovery of stage N3 due to disturbed and inadequate SWS consolidation occurring in the initial sleep cycles.
In our study, 80% of DoA subjects and all SHE patients presented EEG abnormalities during sleep, a perturbing factor which can trigger a phase A of CAP and subsequent arousal instability. In addition, a number of sleep disorders can also promote the unstable NREM sleep background on which DoA and SHE events occur. The triggering role of sleep deprivation ( 2 , 36 ), medications; ( 37 , 38 ), and sleep disordered breathing ( 38 ), (see Figure 4 ), including upper airway resistance syndrome ( 21 ) should be systematically searched for and treated in the presence of parasomnias or sleep-related epileptic episodes. Some authors ( 12 , 39 ) have considered hypersynchronous slow delta activity (HSDA), i.e., bursts of high amplitude slow delta with fronto-central gradient occurring during NREM sleep, as the typical triggering EEG pattern of DoA ( Figure 1 ). However, HSDA presents a clear overlap with CAP subtype A1 ( 21 ) and the unstable background may lead to stage N3 vulnerability which promotes the occurrence of motor episodes. According to our results both DoA and SHE patients presented significantly higher levels of SWS instability with respect to healthy sleepers (CAP in N3 being, respectively, +36 and + 46%). Similar results were described in a cohort of adult DoA patients and designated as “SWS fragmentation index” ( 34 ). In this dynamic landscape, subtypes A3 ( 21 ), which are the longest A phases of CAP ( 40 ) and are increased in sleepwalkers ( 21 ), probably play a weakening effect on N3 consolidation and are involved in the typical sleep-state dissociation when SWS and arousals coexist.
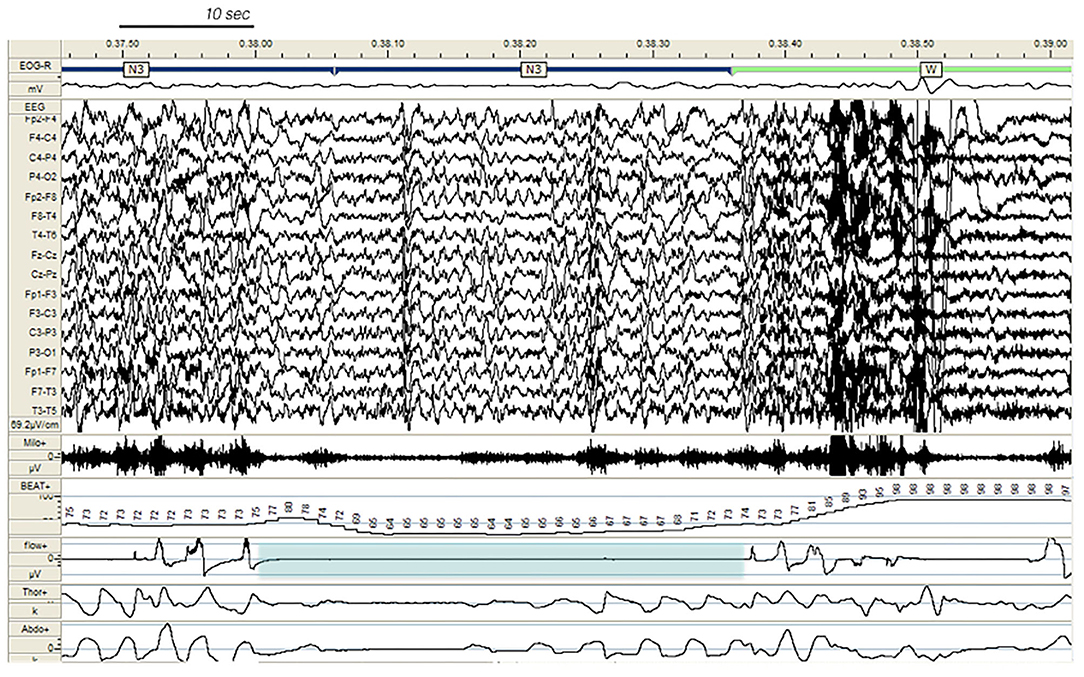
Figure 4 . Paroxysmal arousal in a patient with SHE and untreated obstructive sleep apnea (not included in the study), beginning during stage N3 and triggered by a respiratory event (blue shadow). Bursts of generalized epileptiform discharges, slightly prevailing over the left hemisphere, which can be appreciated throughout the recording, are increased in amplitude during arousals. EEG sensitivity 69.2 mV/cm; Milo +, chin electromyography; BEAT, heart frequency; flow+, airflow thermistor; Thor+, plethysn1ography thoracic band; Abdo+, plethysmography abdominal band.
NREM/REM Imbalance
Our SHE and DoA recordings were characterized by a significant reduction of REM sleep suggesting an imbalanced control of REM-on and REM-off forces. REM and NREM sleep are mutually linked and regulated by a reciprocal interaction ( 41 ). REM sleep also attenuated the spreading of EEG paroxysms, acting as a protective stage towards propagation of epileptiform discharges ( 42 ) ( Figure 3 ).
Neurophysiological and neuroimaging studies in subjects with DoA have provided evidence of abnormal brain functioning not only during SWS but also during REM sleep ( 43 ). Quantitative EEG analysis carried out in sleepwalkers during non-sleepwalking nights shows that the absolute power of delta waves is significantly lower in sleepwalkers compared to controls during the first NREM-REM cycle ( p = 0.03) and a very important trend ( p = 0.059) is noted for the second sleep cycle ( 21 ). REM sleep is frequently curtailed also in adults ( 44 ) and children ( 45 ) suffering from sleep-related epilepsy. When antiepileptic medication attenuates the occurrence of major episodes in SHE patients, sleep cycles recover a physiological architecture and a normal REM-latency due to a more solid sleep structure especially in the first part of the night ( 30 ). In contrast, the A3 phases, which are also physiologically involved in the ultradian process of sleep ( 46 ) and show increased amounts in both untreated SHE ( 14 ) and DoA ( 21 , 34 ), are unaffected by antiepileptic therapy ( 30 ).
Clinical Manifestations: The Role of Sleep Staging and Arousal Instability
In line with previous reports, the commonest motor manifestation in our DoA group was represented by SAM (94%). A v-PSG assessment of 334 DoA episodes documented that 84% were SAM, 10% were RAM and 5% were CAM ( 47 ). In SHE patients, the majority of NREM seizures arose from stage N2, but most major attacks showed a preferential occurrence during SWS (57%). The different distribution of motor episodes across the night and within the NREM stages is a widely accepted issue ( 6 , 35 , 48 ). According to a recent study, the occurrence of at least one minor event during stage N3 is highly suggestive for DoA, while the occurrence of at least one major event outside stage N3 is highly suggestive for SHE ( 47 ). However, in the same study, the number of major events in stage N3 per subject coincided in both DoA and SHE patients ( 47 ), suggesting that sleep staging is not a major element for the differential diagnosis ( 8 ).
Probably, a different modulation of NREM stages on major and minor motor events in DoA and SHE patients is a more plausible statement. A close relation between MME and arousal fluctuations is also a consolidated issue ( 20 ). Therefore, besides classifying pathologies (DoA and SHE) according to the stage-distribution of nocturnal episodes, perhaps a greater attention on the unstable balance between arousal-promoting and sleep-promoting forces may provide additional informations regardless of the ongoing sleep stage.
Central Pattern Generators (CPG)
As the great majority of nocturnal episodes were simple arousal movements (94% in the DoA group) or minor motor events (75% in the SHE group), the recorded behaviors probably expressed the fragments of a hierarchical continuum characterized by increasing intensity, complexity, and duration of the nocturnal episodes. The motor patterns which are already written in the brain codes need a window of arousal to become visibly apparent ( 40 ). Encoded CPG seem to be involved in the genesis of involuntary movements during sleep ( 49 ). The CPG system is composed of spinal and brainstem networks regulated by a supraspinal circuitry and produces coordinated and stereotyped locomotor movements such as walking or swimming, important for survival ( 50 ). The repetitive arousals of CAP activate these cortico-subcortical-spinal pathways, facilitating or releasing sleep-related behaviors such as NREM parasomnia or seizures. Regardless of the sleep disturbance, the arousal-induced activation of the CPG system generates stereotyped motor manifestations that often cause difficulties in the differential diagnosis between NREM parasomnias and SHE ( Figure 5 ). It must be recalled that even highly stereotyped minor motor events can occur in the absence of an epileptiform discharge ( 7 ). In other words, CAP can be a common denominator of an arousal-related motor dishinibition whether or not epileptic in origin ( 51 ). The behavioral outcome relies on a number of factors including the local cerebral regions and activated networks.
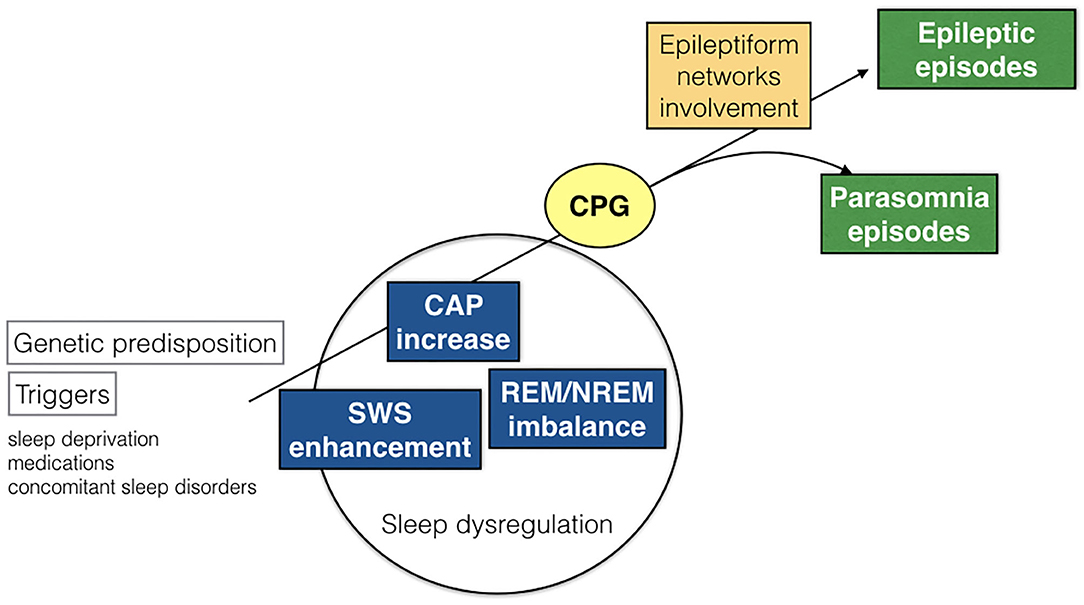
Figure 5 . Schematic representation of pathogenetic commonalities between DoA and SHE. CAP, cyclic alternating pattern; SWS, slow wave sleep; CPG, central pattern generators.
NREM vs. REM Parasomnias
These findings corroborate the impressive overlap between DoA and SHE in terms of semiology, EEG features, sleep patterns, cerebral regions and common triggers. Even if we are dealing with two distinct conditions, the blurred boundaries between them support the possibility of a continuum between DoA and SHE. The imbalance between arousal and sleep forces may entail variable motor manifestations determined by multiple factors, i.e., genetic predisposition, involvement and thresholds of specific brain areas and neural circuits, CPG, paroxysmal discharges, and activation of epileptic networks. However, the numerous commonalities suggest that DoA and SHE share basic NREM sleep-related pathogenetic mechanisms. Accordingly, the high amounts of CAP rate found in both DoA and SHE patients are opposed to the reduced levels of CAP rate in REM sleep parasomnias ( 52 ).
Arousal Models Commonalities for DoA and SHE
So far three fascinating pathophysiological hypothesis focusing on arousal system functioning have been formulated to explain commonalities between SHE and DoA, respectively, named the “liberation,” “dissociation,” and “pathological” arousal models ( 53 ). The first focuses on functional de-inactivation of frontal lobe by subcortical nuclei (CPG) due to variable external or internal stimuli ( 54 ); the second recognizes the simultaneous mixed sleep and awake state existence as a major determinant for clinical manifestations ( 55 , 56 ); the third harmonizes the previous models, assuming the existence of a gain-of-function of frontal cortical acetilcholine receptors in both SHE and DoA, explaining their semiological differences according to underlying facilitatory circumstances ( 53 ).
Limitations and Unanswered Questions
Despite these consistent clues, a number of questions need to be addressed. Is the strong convergence between DoA and SHE basically due to our clinical difficulty to distinguish single sleep disorders? Are we using adequate tools to investigate behavioral manifestations during sleep? Are we dealing with a two-faced entity, which can offer one of the two sides of the coin even in the same patient and in the same night? Does it really matter to establish that a nocturnal motor event occurs in stage N2 or stage N3 if the common background is an unstable NREM sleep? Why are antiepileptic drugs often effective in the treatment of DoA episodes? Challenging issues, which represent intrinsic weaknesses of the study but encourage further investigations on the distinctive features and common nature of DoA and SHE. Another limitation of our investigation could be attributed to its retrospective nature, to the relatively small sample and to partial exploitation of published data ( 14 ). However, the entire framework–recruitment and recording–was carried out in a homogeneous setting (Sleep Disorders Center at Parma University Hospital) and scoring was completed by the same sleep team. Finally our SHE cohort included 10/40 patients with a positive family history for NREM sleep parasomnia in their first-degree members. Even if none of the included SHE patients presented nocturnal DoA-like manifestations nor other known sleep disorders, previous studies demonstrated ( 6 , 57 ) that epileptic and parasomnic events frequently coexist and their semiological differentiation, especially when minor motor episodes prevail, is often a challenging issue ( 10 ) which requires accurate evaluation ( 3 ).
Conclusions and Perspectives
SHE is considered a rare disease, with a crude prevalence among adults around 1.8/100.000 ( 58 ), while the prevalence rate of sleepwalking is estimated 5% in children and 1.5% in adults ( 59 ). As a possible explanation of these diverging data, SHE represents the tip of the iceberg acting in the same pathophysiological continuum of DoA, with potential underestimation due to the numerous diagnostic issues described in the present study. A common genetic background shared by DoA and SHE is also hypothesized. Familial aggregation of patients with diagnosed SHE and the higher frequency of arousal parasomnias in SHE probands and their relatives compared with a control population ( 18 , 57 , 60 ) support an intriguing affinity. The involvement of cholinergic pathways has been suggested in abnormal arousal reaction ( 57 , 61 ). In particular, acetilcholine is one the major neurotransmitters of the ascending reticular activating system and nicotinic acetylcholine receptors are widely distributed in the brain and modulate arousal oscillations at cortical and subcortical levels ( 53 , 62 ). Given the clinical and electrophysiological commonalities between SHE and DoA and their frequent overlap in the same patients and families, further research on the cholinergic system and other neurotransmitters involved in the modulation of arousal and sleep is a mandatory challenge ( 63 ).
Recently, a provocative paper highlighted the impressive parallelism between DoA and SHE based on the arousal system's hyperfunction and NREM sleep dissociation states ( 64 ). A brilliant conclusion on the dual nature of DoA and SHE is also available in The Philosophy of Sleep written almost 200 years ago. The author describes “the case of a watchmaker's apprentice who had an attack of sleep-walking every fortnight. In this state, though insensible to all external impressions, he would perform his work with his usual accuracy, and was always astonished, on awaking, at the progress he had made. The paroxysm began with a sense of heat in the epigastrium extending to the head, followed by confusion of ideas and complete insensibility, the eyes remaining open with a fixed and vacant stare. This case, which undoubtedly originated in some diseased state of the brain, terminated in epilepsy” ( 65 ).
Data Availability Statement
The raw data supporting the conclusions of this article will be made available by the authors, under request.
Ethics Statement
The studies involving human participants were reviewed and approved by Comitato Etico dell'area Vasta Emilia Nord (AVEN). The patients/participants provided their written informed consent to participate in this study.
Author Contributions
GB, NB, IT, NA and RC collected the data. GP made statical analysis. CM and LP drafted the manuscript. All authors contributed to the design of the study protocol, critically reviewed the manuscript, and approved its submitted version.
Conflict of Interest
The authors declare that the research was conducted in the absence of any commercial or financial relationships that could be construed as a potential conflict of interest.
1. AASM. International Classification of Sleep Disorders. 3 ed. Darien, IL: American Academy of Sleep Medicine (2014)
Google Scholar
2. Zadra A, Desautels A, Petit D, Montplaisir J. Somnambulism: clinical aspects and pathophysiological hypotheses. Lancet Neurol. (2013) 12:285–94. doi: 10.1016/S1474-4422(12)70322-8
PubMed Abstract | CrossRef Full Text | Google Scholar
3. Loddo G, Sessagesimi E, Mignani F, Cirignotta F, Mondini S, Licchetta L, et al. Specific motor patterns of arousal disorders in adults: a video-polysomnographic analysis of 184 episodes. Sleep Med . (2018) 41:102–9. doi: 10.1016/j.sleep.2017.08.019
4. Montagna P. Nocturnal paroxysmal dystonia and nocturnal wandering. Neurology. (1992) 42 (7 Suppl. 6):61–7.
PubMed Abstract | Google Scholar
5. Oldani A, Zucconi M, Ferini-Strambi L, Bizzozero D, Smirne S. Autosomal dominant nocturnal frontal lobe epilepsy: electroclinical picture. Epilepsia. (1996) 37:964–76. doi: 10.1111/j.1528-1157.1996.tb00534.x
6. Provini F, Plazzi G, Tinuper P, Vandi S, Lugaresi E, Montagna P. Nocturnal frontal lobe epilepsy. A clinical and polygraphic overview of 100 consecutive cases. Brain. (1999) 122 (Pt. 6):1017–31. doi: 10.1093/brain/122.6.1017
7. Terzaghi M, Sartori I, Mai R, Tassi F, Francione S, Cardinale F, et al. Sleep-related minor motor events in nocturnal frontal lobe epilepsy. Epilepsia. (2007) 48:335–41. doi: 10.1111/j.1528-1167.2006.00929.x
8. Tinuper P, Bisulli F, Cross JH, Hesdorffer D, Kahane P, Nobili L, et al. Definition and diagnostic criteria of sleep-related hypermotor epilepsy. Neurology. (2016) 86:1834–42. doi: 10.1212/WNL.0000000000002666
9. Zucconi M, Oldani A, Ferini-Strambi L, Bizzozero D, Smirne S. Nocturnal paroxysmal arousals with motor behaviors during sleep: frontal lobe epilepsy or parasomnia?. J Clin Neurophysiol. (1997) 14:513–22. doi: 10.1097/00004691-199711000-00008
CrossRef Full Text | Google Scholar
10. Vignatelli L, Bisulli F, Provini F, Nalid I, Pittau F, Zaniboni A, et al. Interobserver reliability of video recording in the diagnosis of nocturnal frontal lobe seizures. Epilepsia. (2007) 48:1506–11. doi: 10.1111/j.1528-1167.2007.01121.x
11. Derry CP, Harvey AS, Walker MC, Duncan JS, Berkovic SF. NREM arousal parasomnias and their distinction from nocturnal frontal lobe epilepsy: a video EEG analysis. Sleep. (2009) 32:1637–44. doi: 10.1093/sleep/32.12.1637
12. Jacobson A, Kales A, Lehmann D, Zweizig J. Somnambulism: all-night electroencephalographic studies. Science. (1965) 146:975–7. doi: 10.1126/science.148.3672.975
CrossRef Full Text
13. Halasz P, Terzano M, Parrino L, Bodizs R. The nature of arousal in sleep. J Sleep Res. (2004), 13:1–23. doi: 10.1111/j.1365-2869.2004.00388.x
14. Parrino L, De Paolis F, Milioli G, Gioi G, Grassi A, Riccardi S, et al. Distinctive polysomnographic traits in nocturnal frontal lobe epilepsy. Epilepsia. (2012) 53:1178–84. doi: 10.1111/j.1528-1167.2012.03502.x
15. Kokkinos V, Koupparis AM, Koustopoulos GK. An intra-K-complex oscillation with independent and labile frequency and topography in NREM sleep. Front Hum Neurosci. (2013) 7:163. doi: 10.3389/fnhum.2013.00163
16. Halasz P, Parrino L, Szucs A. Reactive slow waves in sleep underlying several physiological and pathological NREM conditions. Comments on Flamand, et al. Sleep 2018; 41(10). Sleep . (2019) 42:zsy248. doi: 10.1093/sleep/zsy248
PubMed Abstract | CrossRef Full Text
17. Zucconi M, Oldani A, Ferini-Strambi L, Smirne A. Arousal fluctuations in non- rapid eye movement parasomnias: the role of arousal instability. J Clin Neurophysiol. (1995) 12:147–54. doi: 10.1097/00004691-199503000-00005
18. Tinuper P. Parasomnias versus epilepsy: common grounds and a need to change the approach to the problem. Epilepsia. (2007) 48:1033–4. doi: 10.1111/j.1528-1167.2007.01009_5.x
19. Parrino L, Halasz P, Tassinari CA, Terzano MG. CAP, epilepsy and motor events during sleep: the unifying role of arousal. Sleep Med Rev. (2006) 10:267–85. doi: 10.1016/j.smrv.2005.12.004
20. Sforza E, Montagna P, Rinaldi R, Tinuper P, Cerullo A, Cirignotta F, et al. Paroxysmal periodic motor attacks during sleep: clinical and polygraphic features. Electroencephalogr Clin Neurophysiol. (1993) 86:161–6. doi: 10.1016/0013-4694(93)90003-E
21. Guilleminault C, Kirisoglu C, Rosa AC, Lopes MC, Chan A. Sleepwalking, a disorder of NREM sleep instability. Sleep Med. (2006) 7:163–70. doi: 10.1016/j.sleep.2005.12.006
22. Berry RB, Brooks R, Gamaldo C, Harding SM, Llyod RM, Quan SF, et al. AASM scoring manual updates for 2017 (Version 2.4). J Clin Sleep Med. (2017) 13:665–6. doi: 10.5664/jcsm.6576
23. Terzano MG, Parrino L, Sherieri A, Chervin R, Chokroverty S, Guilleminault C, et al. Atlas, rules, and recording techniques for the scoring of cyclic alternating pattern (CAP) in human sleep. Sleep Med. (2001) 2:537–53. doi: 10.1016/S1389-9457(01)00149-6
24. The Jamovi Project 2019 Jamovi . (Version 1.1) [Computer Software]. Retrieved from https://www.jamovi.org
25. Cohen J. Statistical Power Analysis for the Behavioural Sciences. 2nd ed . New York, NY: Academic Press (1988).
26. Scheffer IE, Berkovic S, Capovilla G, Connolly MB, French J, Guilhoto L, et al. ILAE classification of the epilepsies: position paper of the ILAE commission for classification and terminology. Epilepsia. (2017) 58:512–21. doi: 10.1111/epi.13709
27. Terzano MG, Parrino L, Boselli M, Spaggiari MC, Di Giovanni G. Polysomnographic analysis of arousal responses in obstructive sleep apnea syndrome by means of the cyclic alternating pattern. J Clin Neurophysiol. (1996) 13:145–55. doi: 10.1097/00004691-199603000-00005
28. Parrino L, Thomas RJ, Smerieri A, Spaggiari MC, Del Felice A, Terzano MG. Reorganization of sleep patterns in severe OSAS under prolonged CPAP treatment. Clin Neurophysiol. (2005) 116:2228–39. doi: 10.1016/j.clinph.2005.05.005
29. Korkmaz S, Bilecenoglu NT, Aksu M, Yoldas TK. Cyclic alternating pattern in obstructive sleep apnea patients with versus without excessive sleepiness. Sleep Disord . (2018) 2018:8713409. doi: 10.1155/2018/8713409
30. de Paolis F, Colizzi E, Milioli G, Grassi A, Riccardi S, Puligheddu M, et al. Effects of antiepileptic treatment on sleep and seizures in nocturnal frontal lobe epilepsy. Sleep Med. (2013) 14:597–604. doi: 10.1016/j.sleep.2013.02.014
31. Gaudreau H, Joncas S, Zadra A, Montplaisir J. Dynamics of slow-wave activity during the NREM sleep of sleepwalkers and control subjects. Sleep. (2000) 23:755–60. doi: 10.1093/sleep/23.6.1d
32. Guilleminault C, Poyares D, Aftab FA, Palombini L. Sleep and wakefulness in somnambulism: a spectral analysis study. J Psychosom Res. (2001) 51:411–6. doi: 10.1016/S0022-3999(01)00187-8
33. Zadra A, Pilon M, Montplaisir J. Polysomnographic diagnosis of sleepwalking: effects of sleep deprivation. Ann Neurol. (2008) 63:513–9. doi: 10.1002/ana.21339
34. Lopez R, Shen Y, Chenini S, Rassu AL, Evangelista E, Barateau L, et al. Diagnostic criteria for disorders of arousal: a video-polysomnographic assessment. Ann Neurol. (2018) 83:341–51. doi: 10.1002/ana.25153
35. Barros A, Uguccioni G, Salkin-Goux V, Leu-Semenescu S, Dodet P, Arnulf I. Simple behavioral criteria for the diagnosis of disorders of arousal. J Clin Sleep Med. (2020) 16:121–8. doi: 10.5664/jcsm.8136
36. Halász P, Filakovszky J, Vargha A, Bagdy G. Effect of sleep deprivation on spike-wave discharges in idiopathic generalised epilepsy: a 4 x 24 h continuous long term EEG monitoring study. Epilepsy Res. (2002) 51:123–32. doi: 10.1016/S0920-1211(02)00123-7
37. Stallman HM, Kohler M, White J. Medication induced sleepwalking: a systematic review. Sleep Med Rev. (2018) 37:105–13. doi: 10.1016/j.smrv.2017.01.005
38. Crunelli V, Emri Z, Leresche N. Unravelling the brain targets of gamma-hydroxybutyric acid. Curr Opin Pharmacol. (2006) 6:44–52. doi: 10.1016/j.coph.2005.10.001
39. Pilon M, Zadra A, Joncas S, Montplaisir J. Hypersynnchronous delta waves and sonnambulism : brain topography and effects on sleep deprivation. Sleep. (2005) 29:77–84. doi: 10.1093/sleep/29.1.77
40. Parrino L, Boselli M, Spaggiari MC, Smerieri A, Terzano MG. Cyclic alternating pattern (CAP) in normal sleep: polysomnographic parameters in different age groups. Electroencephalogr Clin Neurophysiol. (1998) 107:439–50. doi: 10.1016/S0013-4694(98)00108-4
41. Massaquoi SG, McCarley RW. Extension of the limit cycle reciprocal interaction model of REM cycle control. An integrated sleep control model. J Sleep Res. (1992) 1:138–43. doi: 10.1111/j.1365-2869.1992.tb00027.x
42. McLeod GA, Ghassemi A, Ng MC. Can REM sleep localize the epileptogenic zone? A systematic review and analysis. Front Neurol. (2020) 11:584. doi: 10.3389/fneur.2020.00584
43. Castelnovo A, Lopez R, Proserpio P, Nobili L, Dauvilliers Y. NREM sleep parasomnias as disorders of sleep-state dissociation. Nat Rev Neurol. (2018) 14:470–81. doi: 10.1038/s41582-018-0030-y
44. Bazil CW, Castro LH, Walczak TS. Reduction of rapid eye movement sleep by diurnal and nocturnal seizures in temporal lobe epilepsy. Arch Neurol. (2000) 57:363–8. doi: 10.1001/archneur.57.3.363
45. Chan SY. Sleep architecture and homeostasis in children with epilepsy: a neurodevelopmental perspective. Dev Med Child Neurol. (2020) 62:426–33. doi: 10.1111/dmcn.14437
46. Terzano MG, Parrino L, Smerieri A, Carli F, Nobili L, Donadio S, et al. CAP and arousals are involved in the homeostatic and ultradian sleep processes. J Sleep Res. (2005) 14:359–68. doi: 10.1111/j.1365-2869.2005.00479.x
47. Proserpio P, Loddo G, Zubler F, Ferini-Strambi L, Licchetta L, Bisulli F, et al. Polysomnographic features differentiating disorder of arousals from sleep-related hypermotor epilepsy. Sleep. (2019) 42:zsz166. doi: 10.1093/sleep/zsz166
48. Silvestri R, Walter AS. Rhythmic movements in sleep disorders and in epileptic seizures during sleep. Sleep Sci Pract. (2020) 4:5 doi: 10.1186/s41606-020-0042-6
49. Tassinari CA, Gardella E, Meletti S, Rubboli G. The neuroethological interpretation of motor behaviours in “nocturnal-hyperkinetic-frontal seizures”: emergence of ‘innate’ motor behaviours and role of central pattern generators. In: Zifkin B, editor. Frontal Seizures and Epilepsies in Children . Montrouge: John Libbey Eurotext (2003). p. 43–8.
50. Guertin P. Central pattern generator for locomotion: anatomical, physiological, and pathophysiological considerations. Front Neurol. (2013) 3:183. doi: 10.3389/fneur.2012.00183
51. Terzaghi M, Manni R. Arousal gating motor events in NFLE–a window on the boundary between physiology and pathology. Sleep Med. (2012) 13:215–6. doi: 10.1016/j.sleep.2012.01.007
52. Melpignano A, Parrino L, Santamaria J, Gaig C, Trippi I, Serradell M, et al. Isolated rapid eye movement sleep behavior disorder and cyclic alternating pattern: is sleep microstructure a predictive parameter of neurodegeneration? Sleep. (2019) 42:zsz142. doi: 10.1093/sleep/zsz142
53. Halász P, Kelemen A, Szucs A. Physiopathogenetic interrelationship between nocturnal frontal lobe epilepsy and NREM arousal parasomnias. Epilepsy Res Treat. (2012) 2012:312693. doi: 10.1155/2012/312693
54. Tassinari CA, Rubboli G, Gardella E, Cantalupo G, Calandra-Buonaura G, Vedovello M, et al. Central pattern generators for a common semiology in fronto-limbic seizures and in parasomnias. A neuroethologic approach. Neurol Sci. (2005) 26 (Suppl. 3):s225–32. doi: 10.1007/s10072-005-0492-8
55. Terzaghi M, Sartori I, Tassi L, Didato G, Rustioni V, LoRusso G, et al. Evidence of dissociated arousal states during nrem parasomnia from an intracerebral neurophysiological study. Sleep. (2009) 32:409–12. doi: 10.1093/sleep/32.3.409
56. Bassetti C, Vella S, Donati F, Wielepp P, Weder B. SPECT during sleepwalking. Lancet. (2000) 356:484–5. doi: 10.1016/S0140-6736(00)02561-7
57. Bisulli F, Vignatelli L, Naldi I, Licchetta L, Provini F, Plazzi G, et al. Increased frequency of arousal parasomnias in families with nocturnal frontal lobe epilepsy: a common mechanism?. Epilepsia. (2010) 51:1852–60. doi: 10.1111/j.1528-1167.2010.02581.x
58. Vignatelli L, Bisulli F, Giovannini G, Licchetta L, Naldi I, Mostacci B, et al. Prevalence of nocturnal frontal lobe epilepsy in the adult population of Bologna and Modena, Emilia-Romagna region, Italy. Sleep. (2015) 38:479–85. doi: 10.5665/sleep.4514
59. Stallman HM, Kohler M. Prevalence of sleepwalking: a systematic review and meta-analysis. PLoS ONE. (2016) 11:e0164769. doi: 10.1371/journal.pone.0164769
60. Zucconi M. Sleep-related epilepsy. Handb Clin Neurol. (2011) 99:1109–37. doi: 10.1016/B978-0-444-52007-4.00024-2
61. Picard F, Bruel D, Servent D, Saba W, Fruchart-Gaillard C, Schçllhorn-Peyronneau MA, et al. Alteration of the in vivo nicotinic receptor density in ADNFLE patients: a PET study. Brain. (2006) 129:2047–60. doi: 10.1093/brain/awl156
62. Jones BE. Arousal and sleep circuits. Neuropsychopharmacology. (2020) 45:6–20. doi: 10.1038/s41386-019-0444-2
63. Puligheddu M, Melis M, Pillolla G, Milioli G, Parrino L, Terzano GM, et al. Rationale for an adjunctive therapy with fenofibrate in pharmacoresistant nocturnal frontal lobe epilespy. Epilepsia. (2017) 58:1762–70. doi: 10.1111/epi.13863
64. Halász P, Szu cs A. Sleep and epilepsy link by plasticity. Front Neurol. (2020) 11:911. doi: 10.3389/fneur.2020.00911
65. Robert M. Philosophy of Sleep . Glasgow: WR: M'Phun (1834).
Keywords: disorders of arousal, parasomnia, sleep-related hypermotor epilepsy, SHE, cyclic alternating pattern, CAP, sleep microstructure
Citation: Mutti C, Bernabè G, Barozzi N, Ciliento R, Trippi I, Pedrazzi G, Azzi N and Parrino L (2020) Commonalities and Differences in NREM Parasomnias and Sleep-Related Epilepsy: Is There a Continuum Between the Two Conditions? Front. Neurol. 11:600026. doi: 10.3389/fneur.2020.600026
Received: 28 August 2020; Accepted: 19 November 2020; Published: 11 December 2020.
Reviewed by:
Copyright © 2020 Mutti, Bernabè, Barozzi, Ciliento, Trippi, Pedrazzi, Azzi and Parrino. This is an open-access article distributed under the terms of the Creative Commons Attribution License (CC BY) . The use, distribution or reproduction in other forums is permitted, provided the original author(s) and the copyright owner(s) are credited and that the original publication in this journal is cited, in accordance with accepted academic practice. No use, distribution or reproduction is permitted which does not comply with these terms.
*Correspondence: Liborio Parrino, liborio.parrino@unipr.it
Disclaimer: All claims expressed in this article are solely those of the authors and do not necessarily represent those of their affiliated organizations, or those of the publisher, the editors and the reviewers. Any product that may be evaluated in this article or claim that may be made by its manufacturer is not guaranteed or endorsed by the publisher.
- Open access
- Published: 17 July 2019
Sleep related hyper motor epilepsy (SHE): a unique syndrome with heterogeneous genetic etiologies
- Francesca Bisulli ORCID: orcid.org/0000-0002-1109-7296 1 , 2 ,
- Laura Licchetta 1 , 2 &
- Paolo Tinuper 1 , 2
Sleep Science and Practice volume 3 , Article number: 3 ( 2019 ) Cite this article
5 Citations
4 Altmetric
Metrics details
Sleep-related hypermotor epilepsy (SHE), formerly known as Nocturnal Frontal Lobe Epilepsy is a focal epilepsy characterized by seizures with complex hyperkinetic automatisms and/or asymmetric tonic/dystonic posturing occurring mostly during sleep. SHE is a rare disease with an estimated minimum prevalence of 1.8/100,000 individuals and represent about 10% of drug-resistant surgical cases. This disorder, though uncommon, is of considerable interest to a broad spectrum of specialists, from child neurologists to neurosurgeons. Distinguishing this condition from non-epileptic paroxysmal behaviour occurring physiologically or pathologically during sleep is often difficult and sometimes impossible on clinical grounds alone, even for experienced epileptologists and sleep physicians. Recognized aetiologies of SHE are heterogeneous and include acquired injuries, genetic causes and structural anomalies such as focal cortical dysplasia. Multiple aetiologies (structural-genetic) are also possible. Non-specific clinical features distinguished different aetiologies even if SHE due to structural lesions usually manifests with early-onset drug-resistant seizures and showed a worse long-term prognosis.
The causative genes for SHE are multiple and encode for proteins involved in different molecular pathways. The cholinergic system and the mTOR pathway are the most relevant. This review will provide an exhaustive overview of the genetic background of SHE.
Sleep-related Hypermotor epilepsy (SHE)
SHE, formerly Nocturnal Frontal Lobe Epilepsy (NFLE), is a focal epilepsy characterized by hyperkinetic seizures occurring predominantly in clusters during non-REM sleep.
This disorder affects individuals of both sexes and any age, with a peak of seizure onset during childhood and adolescence (Scheffer et al. 1994 ; Tinuper et al. 2016 ). A familial form of SHE with autosomal dominant inheritance (ADSHE) has been described. So far, more than 100 families have been identified worldwide (Marini and Guerrini 2007 ; Steinlein 2014 ), but no accurate data concerning the prevalence of ADSHE exist.
The estimated prevalence of non-familial SHE in the adult population is 1.8–1.9 per 100,000 (Vignatelli et al. 2015 ; Vignatelli et al. 2017 ). However, the disorder is likely to be under diagnosed, or in some cases misdiagnosed. Distinguishing this condition from non-epileptic paroxysmal behaviour occurring physiologically or pathologically during sleep is often difficult and sometimes impossible on clinical grounds alone, even for experienced epileptologists and sleep physicians. As a result, misdiagnosis is common and patients may be denied effective treatments or treated inappropriately, leading to long-term side effects and the social consequences of erroneous epilepsy diagnosis (e.g., impacts on driver’s licence).
Most patients show a good response to the pharmacological treatment, low doses of carbamazepine at bedtime being the first choice of therapy. However, about one-third of patients are drug-resistant and only 22% achieved terminal remission after a median 16-year follow-up, most with a remitting pattern from disease onset (Licchetta et al., 2017 ). These data, showing the poor outcome after a long follow-up, possibly explain the reason why SHE has been reported in up to 10% of surgical series (Menghi et al. 2018 ). The surgical outcome seems to be relatively good in this population, especially in patients with positive brain MRI.
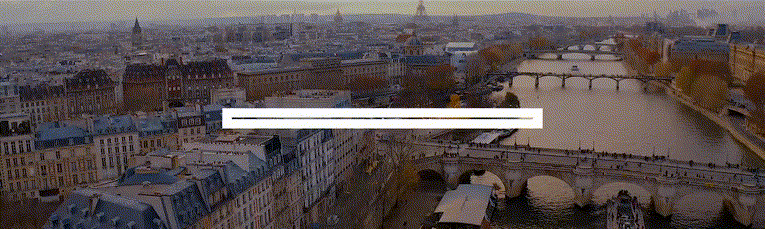
From NPD to SHE
First described in 1981 (Lugaresi and Cirignotta 1981 ), the condition was initially considered a new motor disorder of sleep, namely parasomnia, and the misleading term Nocturnal Paroxysmal Dystonia (NPD) was introduced. Subsequently, similarity of the attacks to those in patients with frontal lobe epilepsy undergoing neurosurgical evaluation (Williamson et al. 1985 ; Waterman et al. 1987 ) and documentation of epileptiform discharges in some patients (Tinuper et al. 1990 ) proved the epileptic origin of the syndrome. The disorder was therefore re-named Nocturnal Frontal Lobe Epilepsy (NFLE). In the following two decades, the clinical boundaries of the disorder were defined, however many controversial issues highlighted the need to change the nomenclature (Tinuper and Bisulli 2017 ). In 2014, a Consensus Conference was held in Bologna, Italy (Tinuper et al. 2016 ). Experts in the field discussed the spectrum of NFLE and produced a final Consensus Statement based on a rigorous protocol addressing nomenclature, electro-clinical definition, diagnostic criteria with levels of certainty supported by available evidence, aetiology, and research needs. The Consensus Conference highlighted three critical issues justifying the change of nomenclature. First, the term nocturnal was considered misleading because it implies a chronobiological pattern of seizure occurrence, whereas evidence indicates that seizure occurrence in sleep is the most important characteristic rather than the time of day. Second, the term frontal lobe is not always appropriate because the characteristic seizures may also arise from extra-frontal areas (Proserpio et al. 2011 ). Third, the term NFLE did not specify the typical clinical semiology involved, which consists primarily of hyperkinetic seizures (Tinuper et al. 2016 ). For all these reasons the term Sleep related Hypermotor Epilepsy (SHE) has been proposed to replace NFLE.
Clinical features
Seizures in SHE are usually brief (< 2 min in duration), with an abrupt onset and offset and with stereotyped motor patterns. The hypermotor semiology is the primary clinical pattern of the seizures, characterized by hyperkinetic features possibly associated with asymmetric tonic/dystonic posturing with or without head/eye deviation. In a minority of cases the asymmetric dystonic posturing is the unique feature of the seizures (Vignatelli et al. 2015 ). Seizures typically show variable complexity and duration varying from brief stereotyped sudden arousals from sleep (paroxysmal arousals or minor motor events) to more complex dystonic-dyskinetic seizures and, more rarely, prolonged ambulatory behavior known as “epileptic nocturnal wandering” (Montagna 1992 ; Provini et al. 1999 ; Nobili et al. 2003 ; Terzaghi et al. 2008 ). Retained awareness during seizures is common and affected individuals may report a distinct aura. Seizures occur typically in cluster during (non-REM) sleep even if episodes during active wakefulness may rarely occur during the patient’s lifetime.
Seizure frequency in SHE patients can be very high, ranging from one to more than 50 attacks per night (Scheffer et al. 1994 ; Provini et al. 1999 ) and paroxysmal arousals or minor motor events may be even more frequent. SHE patients can complain of nocturnal sleep disruption with spontaneous midsleep awakening, sleep inertia in the morning, tiredness at awakening and excessive sleepiness (Peled and Lavie 1986 ; Maccario and Lustman 1990 ; Schwalen and Jorg 1998 ; Zucconi et al. 2000 ; Alanis-Guevara et al. 2005 ) impacting negatively on their quality of life. Although daytime sleepiness-related symptoms could be common in SHE patients, the frequency of excessive daytime sleepiness did not statistically differ compared to controls (Vignatelli et al. 2006 ).
Neurophysiological features
Background activity is usually normal. Interictal EEG is normal in about half cases (Licchetta et al. 2017 ) or may demonstrate rare epileptiform abnormalities, enhanced by sleep deprivation and occurring mainly during sleep (Menghi et al. 2018 ). The ictal scalp EEG may be normal or may only demonstrate movement artifacts. Epileptiform abnormalities, rhythmic slow activity or diffuse background flattening over frontal areas are seen in 50–60% of cases (Licchetta et al. 2017 ).
Scalp EEG and invasive intracranial stereo-electroencephalography recordings (SEEG) documented a frontal lobe origin of seizures in most cases (Nobili et al. 2007 ; Rheims et al. 2008 ). However, in up to 20% of drug resistant cases the ictal discharges may arise from various extra-frontal areas including temporal (Nobili et al. 2004 ; Vaugier et al. 2009 ), insulo-opercular (Ryvlin et al. 2006 ; Dobesberger et al. 2008 ; Nguyen et al. 2009 ; Proserpio et al. 2011 ) and parietal (Montavont et al. 2013 ; Gibbs et al. 2016 ) cortices, then propagating to the frontal cortex and resulting in hypermotor seizures.
Diagnostic criteria
The clinical history and clinicffttal semiology of the attacks are the main criteria to establish the diagnosis, as both interictal and ictal EEG may be uninformative (Scheffer et al. 1994 ; Oldani et al. 1998 ; Nobili et al. 2007 ; Licchetta et al. 2017 ). Three categories for the diagnosis with different levels of certainty have been proposed: 1) Witnessed (possible), based on the description of the core clinical features, as provided by an eye-witness; 2) Video Documented (clinical), based on the evaluation of at least 1 entire (preferably 2) video recorded hyperkinetic episode, confirmed to be typical by witness; 3) Video-EEG documented (confirmed), requiring the video-polygraphic recording of stereotyped events (one or two) and ictal or interictal epileptiform abnormalities (Tinuper et al. 2016 ).
Etiology is unknown in the majority of patients. Recognized etiologies of SHE are heterogeneous and include acquired injuries, genetic causes and structural anomalies such as focal cortical dysplasia (FCD). Multiple etiologies (structural-genetic) are also possible. Non-specific clinical features distinguished different etiologies (Tinuper et al. 2016 ) even if SHE due to structural lesions (FCD) usually manifests with early-onset drug-resistant seizures (Nobili et al. 2009 ) and showed a worse long-term prognosis (Licchetta et al. 2017 ). In these cases, epilepsy surgery and removal of the epileptogenic zone could represent a highly effective treatment option (Nobili et al. 2007 ).
Most patients (86%) are sporadic cases, while 14% reported a family history for epilepsy with only 5% of cases showing a clear-cut autosomal dominant pattern of inheritance, i.e. ADSHE (Licchetta et al. 2017 ). So far ADSHE has been associated with mutations in several genes, encoding proteins involved in different biological pathways. Although the diverse underlying aetiologies and networks involved in its pathogenesis, according to the current state of knowledge, it is considered a single syndrome, defined by clinical manifestations (i.e. hypermotor seizures) resulting from presumed shared downstream mechanisms occurring during sleep/wake oscillation changes. In clinical practice it is most helpful to consider SHE as a single syndrome because it requires a specific diagnostic work-up and therapeutic approach e (Tinuper et al. 2016 ).
Genetics SHE
SHE is the first epilepsy syndrome in which a genetic aetiology was documented. It is also the first the first epilepsy channelopathy described, as it was initially related to mutations in genes coding for subunits of the neuronal nicotinic acetylcholine receptor (nAChR), CHRNA4 , CHRNB2 and CHRNA2 (Steinlein et al. 1995 ).
Subsequently, SHE has been associated with mutations in several other genes, encoding proteins involved in different biological pathways, such as, CRH , KCNT1 , DEPDC5, NPRL2, NPRL3 and PRIMA1 .
Inherited SHE usually shows an autosomal dominant pattern of transmission, except for a single reported family mutated in PRIMA1 , showing an autosomal recessive inheritance (Hildebrand et al. 2015 ).
Ach receptor genes
In 1994, Scheffer et al. (Scheffer et al. 1994 ) described the first large Australian family with SHE inherited in an autosomal dominant manner and named this condition Autosomal Dominant Nocturnal Frontal Lobe Epilepsy (ADNFLE). In this family, SHE had been misdiagnosed as a sleep disorder in many affected members.
Marked variations in severity have been observed amongst different members. Further molecular genetic studies established linkage to chromosome 20q13.2– q13.13 in some families (Phillips et al. 1995 ), leading to the subsequent identification of the causative role of the gene coding for the α 4-subunit of the nAChR, CHRNA4 (Cholinergic Receptor Nicotinic Alpha 4 Subunit, MIM *118504) (Steinlein et al. 1995 ). Following the initial recognition, more than a hundred families have been described worldwide (Marini and Guerrini 2007 ) and mutations in two homologous genes, CHRNB2 (Cholinergic Receptor Nicotinic Beta 2 Subunit, MIM *118507) and CHRNA2 (Cholinergic Receptor Nicotinic Alpha 2 Subunit, MIM *118502), encoding the β2 and α2 subunit of the nAChR respectively, have been identified (De Fusco et al. 2000 ; Aridon et al. 2006 ). The phenotype produced by mutations of the three nAChR subunit genes is generally indistinguishable (McLellan et al. 2003 ).
By now, 14 different mutations in CHRNA4 , CHRNB2 and CHRNA2 have been reported in 20 ADSHE pedigrees and three sporadic cases, as reported in Table 1 . Overall, they account for less than 20% of SHE/ADSHE cases, reflecting the genetic heterogeneity of the syndrome and the possible role of systems other than the cholinergic one, involved in its pathogenesis (Steinlein et al. 2012 ).
In-vitro analyses of the functional properties of nAChR disclosed a functional gain (i.e. an increase in acetylcholine sensitivity) (Bertrand et al. 2002 ) of mutant receptors associated with ADSHE that may underlie the neuronal network dysfunction responsible for the epileptic seizures. Positron-emission tomography (PET) studies in ADSHE patients suggest hyperactivation of the cholinergic pathway ascending from the brainstem (Picard et al. 2006 ). Cholinergic neurons modulate sleep and arousal at both thalamic and cortical levels and their involvement in sleep-related disorders is plausible, although the pathophysiological mechanism remains elusive.
Interestingly, a high prevalence of NREM arousal parasomnias has been reported not only by SHE probands but also by their healthy relatives (Bisulli et al. 2010 ; Licchetta et al. 2017 ) suggesting a possible common background. The relationship between these disorders is also supported by the neurophysiological and neuro-imaging evidence that the pathway controlling physiologic arousal is impaired in both conditions (Picard et al. 2006 ; Montagna et al. 2008 ; Fedi et al. 2008 ).
Seizure frequency improved in a single patient with refractory ADSHE after nicotine transdermal patches treatment (Willoughby et al. 2003 ).
The favourable effect of nicotine on seizure frequency was also described in 9 out of 22 patients from two European ADSHE families carrying CHRNA4 mutations (Brodtkorb and Picard 2006 ). Considering the role of the cholinergic system in arousal regulatory processes, these observations suggested a possible link between nicotine defect, alteration of arousal regulation and seizures in SHE/ADSHE patients. However, despite the reported positive effect of nicotine in reducing seizure frequency, a case–control family study, did not find a higher tendency to smoke tobacco in SHE patients and their relatives compared to the control cases (Naldi et al. 2013 ).
Recently, Puligheddu and colleagues have shown that fenofibrate, an agonist at peroxisome proliferator-activated receptor alpha (PPARa) acting as a negative modulator of nAChRs, may have beneficial effects both in mutated mouse models of SHE and pharmacoresistant SHE patients (Puligheddu et al. 2017 ). Interestingly, good results with fenofibrate administration have been obtained both in the mutated ( CHRNA2 and CHRNA4 ) and non-mutated SHE patients.
Other mutations besides the ones coding for the nAChR subunits have been reported in both sporadic and ADSHE cases. In 2005, Combi et al. found two new nucleotide variations in the CRH (corticotropin-releasing hormone, MIM *122560) promoter in ADSHE pedigrees and sporadic cases (Combi et al. 2005 ) but these findings were not replicated in larger series. The first one (g.1470G > A) recurred in three ADSHE pedigrees and two patients without family history and it was shown to increase CRH levels. The same change was later identified in two affected siblings of another family, but it was also present in a homozygous status in the healthy father (Combi et al. 2008 ), thus reducing the likelihood that the change is pathogenic. The second one (g.1166G > C) was found only in the index-case of a family (Combi et al. 2005 ) and later recognized as non-causative (Combi et al. 2008 ).
Finally, a novel heterozygous exonic missense change was detected in an additional ADSHE family. In vitro assay in this case showed decreased CRH concentrations (Sansoni et al. 2013 ).
CRH encodes for a neurotransmitter/neuromodulator widely distributed throughout the central nervous system that acts in extrahypothalamic circuits to integrate a multisystem response to stress that controls numerous behaviours such as sleep and arousal (Combi et al. 2005 ). The authors suggested that altered (decreased/increased) CHR levels cause increased susceptibility of seizures through excessive sleep fragmentation and brain hyperexcitability (Combi et al. 2005 ).
Further insight into the genetic background of SHE occurred only from 2012 when, combining genome-wide linkage analysis with novel Next Generation Sequencing (NGS) techniques, Heron and coauthors identified a novel gene for SHE, KCNT1 (Potassium Sodium-Activated Channel Subfamily T Member 1, MIM *608167), encoding a subunit of the sodium-activated potassium channel (Heron et al. 2012 ). Mutations in KCNT1 were detected in three ADSHE families with complete penetrance and a sporadic case, all with early-onset refractory seizures, possible intellectual disability and psychiatric or behavioral problems including psychosis, catatonia and aggression (Heron et al. 2012 ). Co-occurrence of mild malformation of cortical development (MCD), namely histologically-confirmed FCD type I and periventricular nodular heterotopia, have been reported very recently in some individuals (Rubboli et al. 2018 ).
Simultaneously, de novo gain-of-function mutations in KCNT1 were identified in six out of 12 unrelated individuals with Malignant Migrating Focal Seizures of Infancy (MMFSI) (Barcia et al. 2012 ), a rare early onset epileptic encephalopathy characterized by refractory, polymorphous focal seizures and arrest of psychomotor development within the first 6 months of life (Coppola et al. 1995 ).
KCNT1 is expressed in the neurons of the frontal cortex (Bhattacharjee et al. 2002 ) and assemble with KCNT2 to form heterotetrameric channel complexes composed of a small amino-terminal domain, a transmembrane domain containing six segments and a large intracellular carboxy-terminal domain containing tandem regulators of potassium conductance domains and an NAD + binding domain. Its activity contributes to the slow hyperpolarization that follows repetitive firing, regulates the rate of bursting and enhances the accuracy with which action potentials lock incoming stimuli (Bhattacharjee and Kaczmarek 2005 ; Brown et al. 2008 ). All the mutations initially described in both ADSHE and MMFSI were clustered around the regulator of potassium conductance and Nicotinamide Adenine Dinucleotide (NAD+) binding domains, which also interacts with a protein network, including fragile X mental retardation protein. Functional study documented that KCNT1 mutations cause a constitutive hyperactivation of the channel that impairs its gating and suppress its subconductance states whit effect on ion currents and increased amplitude. Moreover, they may also alter the conformation of the C-terminal region and its ability to interact with developmentally relevant proteins (Barcia et al. 2012 ). ADSHE mutations were associated with currents approximately 3-fold larger than wild type, while those associated with MMFSI were about 5-fold greater. These differences in the increased ion current amplitude seemed to explain the diversity on phenotypes associated with KCNT1 mutations (Milligan et al. 2014 ). Some of the variants recurred in several patients, suggesting the presence of mutational “hot spots” in KCNT1 (Møller et al. 2015 ). Specific mutations (p.G288S and p.R398Q) can lead to either ADSHE or MMFSI, even within the same family, indicating that genotype–phenotype correlations are not straightforward (Kim et al. 2014 ; Møller et al. 2015 ).
In the last few years, KCNT1 has been implicated in a wide spectrum of focal/multifocal epilepsies and early onset epileptic encephalopathies, in addition to ADSHE and MMFSI phenotypes (Shimada et al. 2014 ; Møller et al. 2015 ; Ohba et al. 2015 ; Rizzo et al. 2016 ).
Very recently, a new therapeutic approach with quinidine was tested in few drug-resistant epileptic patients carrying KCNT1 genetic mutations, no one fitting SHE phenotype, with conflicting results (Mikati et al. 2015 ; Abdelnoura et al. 2018 ).
Surgical treatment of the three unrelated patients with FCD type I reported was ineffective in two individuals (Engel Class IV) and only ameliorate seizure frequency in one (Engel Class II). The authors postulated that the poor surgical outcome may be due to the diffuse nature of FCD type I or to a wider epileptogenic network caused by germline KCNT1 mutations that sustains seizure propensity after epilepsy surgery (Rubboli et al. 2018 ).
GATOR1-complex genes
In 2013, mutations in DEPDC5 (DEP Domain Containing 5, MIM *614191) were implicated in familial focal epilepsy with variable foci (FFEVF) (Dibbens et al. 2013 ), as well as and in a variable percentage (12.5–37%) of heterogenous familial FEs, including ADSHE (Ishida et al. 2013 ; Picard et al. 2014 ).
In particular, DEPDC5 loss-of-function mutations were found in the 13% of a series of 30 families with ADSHE presentation (Picard et al. 2014 ). Electro-Clinical assessments revealed a higher rate of drug resistance and of daytime seizures compared to classical phenotype (Picard et al. 2014 ). DEPDC5 mutations are associated with both lesional and non-lesional epilepsies, even within the same family. In some individuals, brain MRI disclosed MCD, ranging from FCD to subtle band heterotopia, with the predominant pattern being bottom-of-the-sulcus dysplasia, a variety of FCD type IIb (Scheffer et al. 2014 ) (Fig. 1 a-c; Fig. 2 a-c).
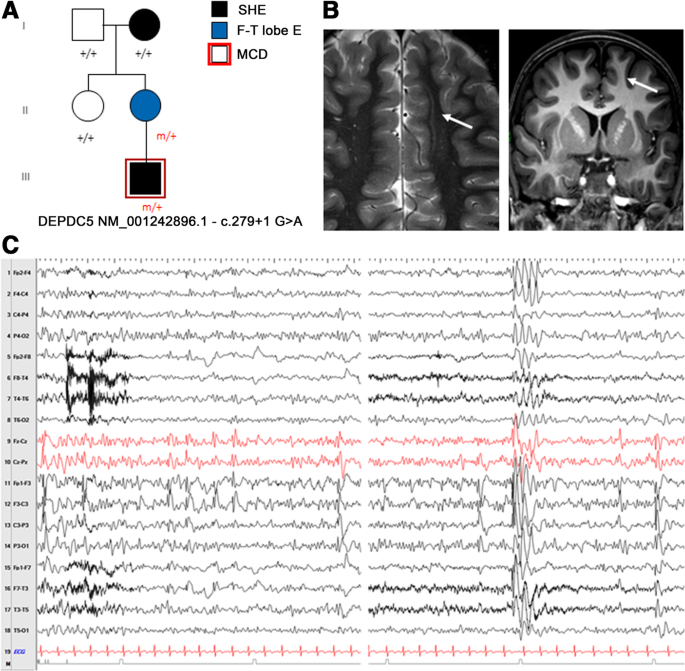
a Pedigree of the family carring the splicing mutation in DEPDC5 c.279 + 1 G > A. Individual III.1 showed Malformation of cortical development at 3 T brain MRI. Previously published in Dibbens et al. 2013 (fam I); Scheffer et al. 2014 (fam C). b Brain MRI of individual III.1. The white arrows point to the unilateral subtle band heterotopia within the white matter adjacent to dysplastic cortex in the left frontal lobe. Blurring of the gray–white matter junction involving part of the cingulate cortex and left frontal cortex was seen. c Interictal EEG of the same individual (III.1) showing frequent spike-wave discharges over the left frontal region (sometimes with phase opposition on F3) enhanced by drowsiness and light sleep, spreading to the ipsilateral and contralateral hemispheres
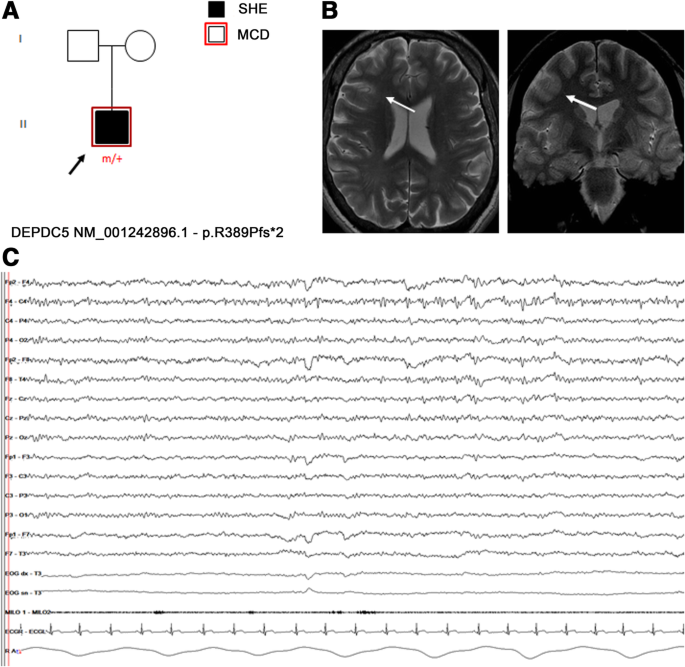
a Pedigree of an isolated SHE patient carring the frameshift DEPDC5 mutation p.R389Pfs*2. Previously published in Baldassari et al., 2019 (Proband 18). b Targeted brain MRI showed a focal increase of cortical thickness with blurring of gray-white matter junction, over the right medium frontal gyrus (white arrows), suggestive of focal cortical dysplasia. c Interictal EEG showing repetitive low-amplitude sharp-waves over the right fronto-central region, enhanced by drowsiness
Subsequently, mutations in NPRL2 (NPR2-like Protein, MIM *607072) (Ricos et al. 2016 ), NPRL3 (Nitrogen Permease Regulator-like 3, MIM *600928) (Korenke et al. 2016 ) have been reported in ADSHE and sporadic cases.
DEPDC5 , NPRL2 and NPRL3 are components of the GATOR1 complex (Gap Activity TOward Rags 1), a negative regulator of the mammalian target of rapamycin (mTOR) complex1 (mTORC1) (Bar-Peled et al. 2013 ). Most of the variants described in these genes are loss of function mutations, with impact on the protein product and consequent hyperactivation of mTORC1 pathway (van Kranenburg et al. 2015 ). In line with this evidence, so far a wide number of germline and somatic (brain-only) mutations of the mTOR-pathway genes have been associated with a range of lesional and non-lesional FEs. With regards to lesional cases, mutations in these genes have been identified in several MCDs and in particular in up to 46% of FCD type IIb (Nakashima et al. 2015 ; Scheffer et al. 2014 ; Lal et al. 2014 ; Martin et al. 2014 ; Scerri et al. 2015 ; D'Gama et al. 2015 ; Baulac et al. 2015 ).
Additional insights into the role of DEDCD5 in FCD-related focal epilepsies derive from the rat model (Marsan et al. 2016 ). The heterozygous rats exhibited an altered cortical neuron excitability and firing patterns and cortical cytomegalic dysmorphic neurons and balloon-like cells strongly expressing phosphorylated rpS6, supporting mTORC1 upregulation. These neuropathological abnormalities are reminiscent of the hallmark brain pathology of human FCD.
These data showed that genetic and structural cause are not mutually exclusive either and, in particular, SHE related to GATOR1-complex genes may have a genetic-structural etiology.
FCDs represent the most common, potentially treatable architectural disorder underlying FE, responsible for up to 42% of drug-resistant cases (Harvey et al. 2008 ). In general, epilepsy surgery is a highly effective curative option in these patients, affording the opportunity to achieve seizure freedom and potential medication withdrawal also with improvements in quality of life, employment rates and school attendance (Wiebe et al. 2001 ). Although mutated patients who have undergone epilepsy surgery are anecdotal, surgery has proved to be curative in cases with MCD clearly detectable with conventional neuroimaging (Baulac et al. 2015 ), suggesting that epileptogenesis is underpinned by a genetically determined, focal cerebral structural lesion, even in the presence of germline mutations. On the other hand the role of surgery in non lesion cases with germline mutation is still controversial/needs to be clarify. In the cited series non lesional cases who underwent surgery had a worse outcome compared with the lesional ones (Baulac et al. 2015 ). Moreover, a SEEG study in a patient carrying DEPDC5 truncating mutation failed to identify a definitive epileptogenic zone (Fig. 3 a-c). These evidences suggest that DEPDC5 germline mutations could play a role in non-lesional, refractory focal epilepsies with multiple independent epileptogenic foci or widespread epileptogenic networks (Ferri et al. 2017 ). According to this hypothesis the presence of germinal mutations in mTOR genes could represent a contraindication not only for surgery but possibly for presurgical invasive procedures (i.e. stereoEEG, corticography). However, given the association of DEPDC5 mutations also with FCD type I lesions (Baulac et al. 2015 ), in these non lesional cases it cannot be excluded the presence of multiple, diffuse subtle dysplastic areas missed by conventional brain MRI.
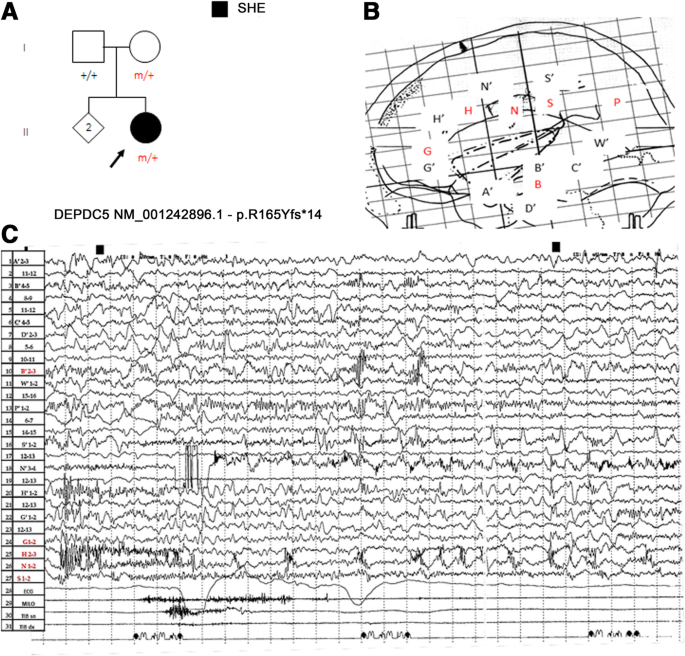
a Pedigree of a 57-year-old female with a frameshift mutation of DEPDC5, p.Arg165Tyrfs*14 inherited by her healthy mother. Previously published in Ferri et al. 2017 . b Stereotactic scheme of the patient who at age 43 years underwent stereoelectroencephalography (SEEG) study with bilateral limbic exploration extended to the inferior parietal lobe. The SEEG exploration shown (lateral view) included 15 intra-cerebral electrodes implanted mainly on the left. The EEG focus area was mainly explored by electrode H. Black letters with the accent (A’, B′, C′, D’, G’, H′, N′, S′, W′) indicates left side; red letters (B, G, H, N, S, P) indicated the right. c SEEG ictal recording showing fast polyspike activity over both the anterior-mid cingulate gyrus preceding a typical nocturnal hypermotor seizure, prevailing on right central-anterior cingulate cortex (H electrode). Note that interictal activity is recorded also in electrodes remote from ictal onset zone. Although the electrical pattern was suggestive for focal cortical dysplasia, tailored brain MRI was unrevealing
A novel missense mutation in the CABP4 gene encoding the neuronal Ca2 + −binding protein 4 (CaBP4) has been found in a Chinese family including 11 individuals diagnosed with ADSHE (Chen et al. 2017 ).
In a two-generation Australian family of Italian origin affected with SHE and ID, Hildebrand and coauthors identified by Whole Exome Sequencing (WES) analysis a homozygous mutation in PRIMA1 (Hildebrand et al. 2015 ). This gene encodes a transmembrane protein that anchors acetylcholinesterase (AChE), the enzyme hydrolyzing Ach to membrane rafts of neurons. The c.93 + 2 T > C mutation identified leads to knockout of PRIMA1, with reduction of AChE and accumulation of acetylcholine at the synapse, as shown in PRIMA1 knockout mice. The authors concluded that, similarly to the gain of function mutations of the genes coding for nAChR subunits, the enhanced cholinergic responses are the likely cause of severe SHE and intellectual disability in this family. However, apart from this single pedigree, this finding has not been replicated yet, as no other mutations were identified in a confirmation cohort of hundreds of SHE probands (Hildebrand et al. 2015 ).
Conclusions
SHE is a heterogeneous genetic syndrome, caused by genes involved in different molecular pathways. Despite a great effort to study the genetic background of SHE, a genetic cause may be recognizable in a very low percentage of sporadic cases and in less than 30% of ADSHE families, with incomplete penetrance (Kurahashi H et al., 2002 ). This is a cumulative estimate coming from studies of different case-series (principally families), each focused on the screening for mutations in a singular SHE gene. A systematic genetic characterization of a population of familial and sporadic patients diagnosed with SHE based on reliable diagnostic criteria is lacking.
Moreover, to date, there are no clear-cut correlations between disease severity, genetic findings and functional effects of the known genetic mutations (Tinuper et al. 2016 ) and further studies focused on genotype-phenotype correlations in SHE are needed. By now, KCNT1 gene mutated SHE patients seem to present a more severe form (Heron et al. 2012 ), whereas mutations in GATOR1-complex genes have been implicated in genetic-structural etiology of SHE.
Although at present genetic testing has limited usefulness in clinical practice, as genetics explain a minority of SHE patients, from a clinical perspective, analysis of SHE-related genes is worth even in isolated cases for whom a genetic aetiology is not primarily considered, because of possible implications for the diagnostic work-up and clinical management. In particular, detection of mutations in GATOR1-complex genes represent a red flag for FCDs, the most common potentially treatable architectural disorder underlying refractory FE. In non-lesional cases carrying pathogenic variants of GATOR1-complex genes, repeated and careful review of targeted, high-resolution imaging is needed to highlight subtle structural abnormalities susceptible of surgery. Moreover, the identification of pathogenic or possibly pathogenic mutations in GATOR1-complex genes, as well as in genes coding proteins acting upstream in the mTOR pathway could have important future therapeutic implications even in mutated patients considered not eligible for surgery. In fact, the development of a novel class of therapies based on mTOR inhibitors, whose prototype is rapamycin, will improve the treatment and prognosis of these patients. Both preclinical and clinical trials using mTOR inhibitors to treat epilepsy, and possibly prevent it, are currently underway (Citraro et al. 2016 ).
Availability of data and materials
Not applicable.
Abbreviations
Acetylcholinesterase
Autosomal dominant nocturnal frontal lobe epilepsy
Autosomal dominant sleep-related hypermotor epilepsy
Focal cortical dysplasia
Focal epilepsy
familial focal epilepsy with variable foci
mammalian Target Of Rapamycin
mammalian Target Of Rapamycin Complex1
neuronal nicotinic ACetylcholine Receptor
Nicotinamide adenine dinucleotide
- Nocturnal frontal lobe epilepsy
Nocturnal paroxysmal dystonia
Positron-emission tomography
Peroxisome proliferator-activated receptor alpha
Stereoelectroencephalography
- Sleep-related hypermotor epilepsy
Whole exome sequencing
Abdelnoura E, et al. Does age affect response to quinidine in patients with KCNT1 mutations? Report of three new cases and review of the literature. Seizure. 2018;55:1–3.
Article Google Scholar
Alanis-Guevara I, et al. Sleep disturbances, socioeconomic status, and seizure control as main predictors of quality of life in epilepsy. Epilepsy Behav. 2005;7:481–5.
Article CAS PubMed Google Scholar
Aridon P, et al. Increased sensitivity of the neuronal nicotinic receptor alpha 2 subunit causes familial epilepsy with nocturnal wandering and ictal fear. Am J Hum Genet. 2006;79:342–50.
Article CAS PubMed PubMed Central Google Scholar
Baldassari S, et al. The landscape of epilepsy-related GATOR1 variants. Genet Med. 2019;21(2):398–408.
Barcia G, et al. De novo gain-of-function KCNT1 channel mutations cause malignant migrating partial seizures of infancy. Nat Genet. 2012;44(11):1255–9.
Bar-Peled L, et al. A tumor suppressor complex with GAP activity for the rag GTPases that signal amino acid sufficiency to mTORC1. Science. 2013;340:1100–6.
Baulac S, et al. Familial focal epilepsy with focal cortical dysplasia due to DEPDC5 mutations. Ann Neurol. 2015;77:675–83.
Bertrand D, et al. How mutations in the nAChRs can cause ADNFLE epilepsy. Epilepsia. 2002;43(Suppl 5):112–22.
Bertrand D, et al. The CHRNB2 mutation I312M is associated with epilepsy and distinct memory deficits. Neurobiol Dis. 2005;20(3):799–804.
Bhattacharjee A, Kaczmarek LKJ. For K+ channels, Na+ is the new Ca2+. Trends Neurosci. 2005;28:422–8.
Bhattacharjee A, et al. Localization of the Slack potassium channel in the rat central nervous system. J Comp Neurol. 2002;454:241–54.
Bisulli F, et al. Increased frequency of arousal parasomnias in families with nocturnal frontal lobe epilepsy: a common mechanism? Epilepsia. 2010;51:1852–60.
Article PubMed Google Scholar
Brodtkorb E, Picard F. Tobacco habits modulate autosomal dominant nocturnal frontal lobe epilepsy. Epilepsy Behav. 2006;9:515–20.
Brown MR, et al. Amino-termini isoforms of the slack K+ channel, regulated by alternative promoters, differentially modulate rhythmic firing and adaptation. J Physiol Lond. 2008;586:5161–79.
Chen Y, et al. A novel mutation of the nicotinic acetylcholine receptor gene CHRNA4 in sporadic nocturnal frontal lobe epilepsy. Epilepsy Res. 2009;83(2–3):152–6.
Chen ZH, et al. Exome sequencing identified a novel missense mutation c.464G>a (p.G155D) in Ca2+−binding protein 4 (CABP4) in a Chinese pedigree with autosomal dominant nocturnal frontal lobe epilepsy. Oncotarget. 2017;8:78940–7.
PubMed PubMed Central Google Scholar
Cho YW, et al. A Korean kindred with autosomal dominant nocturnal frontal lobe epilepsy and mental retardation. Arch Neurol. 2003;60:1625–32.
Cho YW, et al. Autosomal dominant nocturnal frontal lobe epilepsy and mild memory impairment associated with CHRNB2 mutation I312M in the neuronal nicotinic acetylcholine receptor. Epilepsy Behav. 2008;13:361–5.
Citraro R, et al. mTOR pathway inhibition as a new therapeutic strategy in epilepsy and epileptogenesis. Pharmacol Res. 2016;107:333–43.
Combi R, et al. Two new putative susceptibility loci for ADNFLE. Brain Res. 2005;67:257–63.
Article CAS Google Scholar
Combi R, et al. Compound heterozygosity with dominance in the Corticotropin releasing hormone (CRH) promoter in a case of nocturnal frontal lobe epilepsy. J Sleep Res. 2008;17(3):361–2.
Conti V, et al. Nocturnal frontal lobe epilepsy with paroxysmal arousals due to CHRNA2 loss of function. Neurology. 2015;84(15):1520–8.
Coppola G, et al. Migrating partial seizures in infancy: a malignant disorder with developmental arrest. Epilepsia. 1995;36:1017–24.
De Fusco M, et al. The nicotinic receptor beta 2 subunit is mutant in nocturnal frontal lobe epilepsy. Nat Genet. 2000;26:275–6.
D'Gama AM, et al. Mammalian target of rapamycin pathway mutations cause hemimegalencephaly and focal cortical dysplasia. Ann Neurol. 2015;77(4):720–5.
Díaz-Otero F, et al. Autosomal dominant nocturnal frontal lobe epilepsy with a mutation in the CHRNB2 gene. Epilepsia. 2008;49(3):516–20.
Article PubMed CAS Google Scholar
Dibbens LM, et al. Mutations in DEPDC5 cause familial focal epilepsy with variable foci. Nat Genet. 2013;45:546–51.
Dobesberger J, et al. Successful surgical treatment of insular epilepsy with nocturnal hypermotor seizures. Epilepsia. 2008;49:159–62.
Fedi M, et al. Reduced striatal D1 receptor binding in autosomal dominant nocturnal frontal lobe epilepsy. Neurology. 2008;71:795–8.
Ferri L, et al. A stereo EEG study in a patient with sleep-related hypermotor epilepsy due to DEPDC5 mutation. Seizure. 2017;53:51–4.
Gibbs SA, et al. Sleep- related epileptic behaviors and non-REM-related parasomnias: insights from stereo-EEG. Sleep Med Rev. 2016;25:4–20.
Harvey AS, et al. Defining the spectrum of international practice in pediatric epilepsy surgery patients. Epilepsia. 2008;49:146–55.
Heron SE, et al. Missense mutations in the sodium-gated potassium channel gene KCNT1 cause severe autosomal dominant nocturnal frontal lobe epilepsy. Nat Genet. 2012;44:1188–90.
Hildebrand MS, et al. PRIMA1 mutation: a new cause of nocturnal frontal lobe epilepsy. Ann Clin Transl Neurol. 2015;2(8):821–30.
Hirose S, et al. A novel mutation of CHRNA4 responsible for autosomal dominant nocturnal frontal lobe epilepsy. Neurology. 1999;53(8):1749–53.
Hoda JC, et al. Human nocturnal frontal lobe epilepsy: pharmocogenomic profiles of pathogenic nicotinic acetylcholine receptor beta-subunit mutations outside the ion channel pore. Mol Pharmacol. 2008;74(2):379–91.
Ishida S, et al. Mutations of DEPDC5 cause autosomal dominant focal epilepsies. Nat Genet. 2013;45:552–5.
Kim GE, et al. Human slack potassium channel mutations increase positive cooperativity between individual channels. Cell Rep. 2014;9:1661–72.
Korenke GC, et al. Nocturnal frontal lobe epilepsy caused by a mutation in the GATOR1 complex gene NPRL3. Epilepsia. 2016;57(3):e60–3.
Kurahashi H, Hirose S. Autosomal Dominant Nocturnal Frontal Lobe Epilepsy. In: Pagon RA, Adam MP, Ardinger HH, Wallace SE, Amemiya A, Bean LJH, Bird TD, Ledbetter N, Mefford HC, Smith RJH, Stephens K, editors. GeneReviews®. Seattle: University of Washington Seattle; 2002. p. 1993–2017. Available from http://www.ncbi.nlm.nih.gov/books/NBK1169/ [Initial Posting: May 16, 2002; Last Update: March 15, 2018].
Lal D, et al. DEPDC5 mutations in genetic focal epilepsies of childhood. Ann Neurol. 2014;75:788–92.
Leniger T, et al. A new Chrna4 mutation with low penetrance in nocturnal frontal lobe epilepsy. Epilepsia. 2003;44(7):981–5.
Licchetta L, et al. Sleep-related hypermotor epilepsy: long-term outcome in a large cohort. Neurology. 2017;88(1):70–7.
Article PubMed PubMed Central Google Scholar
Liu H, et al. The identification of a novel mutation of nicotinic acetylcholine receptor gene CHRNB2 in a Chinese patient: its possible implication in non-familial nocturnal frontal lobe epilepsy. Epilepsy Res. 2011;95(1–2):94–9.
Lugaresi E, Cirignotta F. Hypnogenic paroxysmal dystonia: epileptic seizure or a new syndrome? Sleep. 1981;4(2):129–38.
Maccario M, Lustman LI. Paroxysmal nocturnal dystonia presenting as excessive daytime somnolence. Arch Neurol. 1990;47:291–4.
Marini C, Guerrini R. The role of the nicotinic acetylcholine receptors in sleep-related epilepsy. Biochem Pharmacol. 2007;74:1308–14.
Marsan E, et al. Depdc5 knockout rat: a novel model of mTORopathy. Neurobiol Dis. 2016;89:180–9.
Martin C, et al. A recurrent mutation in DEPDC5 predisposes to focal epilepsies in the French-Canadian population. Clin Genet. 2014;86:570–4.
McLellan A, et al. Phenotypic comparison of two Scottish families with mutations in different genes causing autosomal dominant nocturnal frontal lobe epilepsy. Epilepsia. 2003;44:613–7.
Menghi V, et al. Sleep-related hypermotor epilepsy: prevalence, impact and management strategies. Nat Sci Sleep. 2018;10:317–26.
Mikati MA, et al. Quinidine in the treatment of KCNT1-positive epilepsies. Ann Neurol. 2015;78(6):995–9.
Milligan CJ, et al. KCNT1 gain of function in 2 epilepsy phenotypes is reversed by quinidine. Ann Neurol. 2014;75:581–90.
Møller RS, et al. Mutations in KCNT1 cause a spectrum of focal epilepsies. Epilepsia. 2015;56(9):e114–20.
Article PubMed PubMed Central CAS Google Scholar
Montagna P. Nocturnal paroxysmal dystonia and nocturnal wandering. Neurology. 1992;42:61–7.
CAS PubMed Google Scholar
Montagna P, et al. Nocturnal epileptic seizures versus the arousal parasomnias. Somnologie. 2008;12:25–37.
Montavont A, et al. Hypermotor seizures in lateral and mesial parietal epilepsy. Epilepsy Behav. 2013;28:408–12.
Nakashima M, et al. Somatic mutations in the MTOR gene cause focal cortical dysplasia type IIb. Ann Neurol. 2015;78:375–86.
Naldi I, et al. Tobacco habits in nocturnal frontal lobe epilepsy. Epilepsy Behav. 2013;26:114–7.
Nguyen DK, et al. Revisiting the role of the insula in refractory partial epilepsy. Epilepsia. 2009;50:2599–604.
Nobili L, et al. Nocturnal frontal lobe epilepsy: intracerebral recordings of paroxysmal motor attacks with increasing complexity. Sleep. 2003;26:883–6.
PubMed Google Scholar
Nobili L, et al. Sleep-related hyperkinetic seizures of temporal lobe origin. Neurology. 2004;62:482–5.
Nobili L, et al. Surgical treatment of drug-resistant nocturnal frontal lobe epilepsy. Brain. 2007;130:561–73.
Nobili L, et al. Taylor’s focal cortical dysplasia increases the risk of sleep-related epilepsy. Epilepsia. 2009;50:2599–604.
Ohba C, et al. De novo KCNT1 mutations in early-onset epileptic encephalopathy. Epilepsia. 2015;56(9):e121–8.
Oldani A, et al. Autosomal dominant nocturnal frontal lobe epilepsy: a video-polysomnographic and genetic appraisal of 40 patients and delineation of the epileptic syndrome. Brain. 1998;121:205–23.
Peled R, Lavie P. Paroxysmal awakenings from sleep associated with excessive daytime somnolence: a form of nocturnal epilepsy. Neurology. 1986;36:95–8.
Phillips HA, et al. Localization of a gene for autosomal dominant nocturnal frontal lobe epilepsy to chromosome 20q13.2. Nat Genet. 1995;10:117–8.
Phillips HA, et al. A de novo mutation in sporadic nocturnal frontal lobe epilepsy. Ann Neurol. 2000;48(2):264–7.
Phillips HA, et al. CHRNB2 is the second acetylcholine receptor subunit associated with autosomal dominant nocturnal frontal lobe epilepsy. Am J Hum Genet. 2001;68(1):225–31.
Picard F, et al. Alteration of the in vivo nicotinic receptor density in ADNFLE patients: a PET study. Brain. 2006;129:2047–60.
Picard F, et al. DEPDC5 mutations in families presenting as autosomal dominant nocturnal frontal lobe epilepsy. Neurology. 2014;82:2101–6.
Proserpio P, et al. Insular-opercular seizures manifesting with sleep-related paroxysmal motor behaviors: a stereo-EEG study. Epilepsia. 2011;52:1781–91.
Provini F, et al. Nocturnal frontal lobe epilepsy. A clinical and polygraphic overview of 100 consecutive cases. Brain. 1999;122:1017–31.
Puligheddu M, et al. Rationale for an adjunctive therapy with fenofibrate in pharmacoresistant nocturnal frontal lobe epilepsy. Epilepsia. 2017;58:1762–70.
Rheims S, et al. Analysis of clinical patterns and underlying epileptogenic zones of hypermotor seizures. Epilepsia. 2008;49:2030–40.
Ricos MG, et al. Mutations in the mammalian target of rapamycin pathway regulators NPRL2 and NPRL3 cause focal epilepsy. Ann Neurol. 2016;79:120–31.
Rizzo F, et al. Characterization of two de novo KCNT1 mutations in children with malignant migrating partial seizures in infancy. Mol Cell Neurosci. 2016;72:54–63.
Rozycka A, et al. Evidence for S284L mutation of the CHRNA4 in a white family with autosomal dominant nocturnal frontal lobe epilepsy. Epilepsia. 2003;44(8):1113–7.
Rubboli G, et al. Mild malformations of cortical development insleep-related hypermotor epilepsy due to KCNT1 mutations. Ann Clin Transl Neurol. 2018;6(2):386–91.
Ryvlin P, Minotti L, Demarquay G, et al. Nocturnal hypermotor seizures, suggesting frontal lobe epilepsy, can originate in the insula. Epilepsia. 2006;47:755–65.
Sáenz A, et al. Autosomal dominant nocturnal frontal lobe epilepsy in a Spanish family with a Ser252Phe mutation in the CHRNA4 gene. Arch Neurol. 1999;56(8):1004–9.
Sansoni V, et al. A de novo mutation in an Italian sporadic patient affected by nocturnal frontal lobe epilepsy. J Sleep Res. 2012;21:352–3.
Sansoni V, et al. Functional characterization of a CRH missense mutation identified in an ADNFLE family. PLoS One. 2013;8(4):e61306.
Scerri T, et al. Familial cortical dysplasia type IIA caused by a germline mutation in DEPDC5. Ann Clin Transl Neurol. 2015;2(5):575–80.
Scheffer IE, et al. Autosomal dominant frontal epilepsy misdiagnosed as sleep disorder. Lancet. 1994;343:515–7.
Scheffer IE, et al. Mutations in mammalian target of rapamycin regulator DEPDC5 cause focal epilepsy with brain malformations. Ann Neurol. 2014;75:782–7.
Schwalen S, Jorg J. Day-time fatigue in frontal lobe epilepsy with primarily sleep-related seizures. A case report. Nervenarzt. 1998;69:166–70.
Shimada S, et al. A novel KCNT1 mutation in a Japanese patient with epilepsy of infancy with migrating focal seizures. Hum Genome Var. 2014;1:14027.
Steinlein OK. Genetic heterogeneity in familial nocturnal frontal lobe epilepsy. Prog Brain Res. 2014;213:1–15.
Steinlein OK, et al. A missense mutation in the neuronal nicotinic acetylcholine receptor α4 subunit is associated with autosomal dominant nocturnal frontal lobe epilepsy. Nat Genet. 1995;11:201–3.
Steinlein OK, et al. An insertion mutation of the CHRNA4 gene in a family with autosomal dominant nocturnal frontal lobe epilepsy. Hum Mol Genet. 1997;6(6):943–7.
Steinlein OK, et al. Independent occurrence of the CHRNA4 Ser248Phe mutation in a Norwegian family with nocturnal frontal lobe epilepsy. Epilepsia. 2000;41(5):529–35.
Steinlein OK, et al. Mutations in familial nocturnal frontal lobe epilepsy might be associated with distinct neurological phenotypes. Seizure. 2012;21:118–23.
Terzaghi M, et al. Coupling of minor motor events and epileptiform discharges with arousal fluctuations in NFLE. Epilepsia. 2008;49:670–6.
Tinuper P, Bisulli F. From nocturnal frontal lobe epilepsy to sleep-related Hypermotor epilepsy: a 35-year diagnostic challenge. Seizure. 2017;44:87–92.
Tinuper P, et al. Nocturnal paroxysmal dystonia with short-lasting attacks: three cases with evidence for an epileptic frontal lobe origin of seizures. Epilepsia. 1990;31(5):549–56.
Tinuper P, et al. Definition and diagnostic criteria of sleep-related hypermotor epilepsy. Neurology. 2016;86:1834–42.
van Kranenburg M, et al. Preliminary functional assessment and classification of DEPDC5 variants associated with focal epilepsy. Hum Mutat. 2015;36(2):200–9.
Vaugier L, et al. Neural networks underlying hyperkinetic seizures of “temporal lobe” origin. Epilepsy Res. 2009;86:200–8.
Vignatelli L, et al. Excessive daytime sleepiness and subjective sleep quality in patients with nocturnal frontal lobe epilepsy: a case-control study. Epilepsia. 2006;47(suppl 5):73–7.
Vignatelli L, et al. Prevalence of nocturnal frontal lobe epilepsy in the adult population of Bologna and Modena, Emilia-Romagna region, Italy. Sleep. 2015;38:479–85.
Vignatelli L, et al. Prevalence of nocturnal frontal lobe epilepsy in the adult population of Bologna and Modena, Emilia-Romagna region. Italy Sleep. 2017;1(2):40.
Google Scholar
Wang MY, et al. A novel mutation of the nicotinic acetylcholine receptor gene CHRNA4 in a Chinese patient with non-familial nocturnal frontal lobe epilepsy. Epilepsy Res. 2014;108(10):1927–31.
Waterman K, et al. An epileptic syndrome caused by mesial frontal lobe seizure foci. Neurology. 1987;37(4):577–82.
Wiebe S, et al. A randomized, controlled trial of surgery for temporal-lobe epilepsy. N Engl J Med. 2001;345:311–8.
Williamson PD, et al. Complex partial seizures of frontal lobe origin. Ann Neurol. 1985;18(4):497–504.
Willoughby JO, et al. Nicotine as an antiepileptic agent in ADNFLE: an N-of-one study. Epilepsia. 2003;44(9):1238–40.
Zucconi M, et al. The macrostructure and microstructure of sleep in patients with autosomal dominant nocturnal frontal lobe epilepsy. J Clin Neurophysiol. 2000;17:77–86.
Download references
Acknowledgements
We would like to acknowledge all our collaborators of Bellaria Hospital, Bologna, especially Dr. Federica Pondrelli and Dr. Toni Francesco. We are also grateful to all the EEG technicians of our staff, in particular Francesco Mignani.
Author information
Authors and affiliations.
IRCCS Istituto delle Scienze Neurologiche di Bologna, Bellaria Hospital, Via Altura 3, Bologna, Italy
Francesca Bisulli, Laura Licchetta & Paolo Tinuper
Department of Biomedical and Neuromotor Sciences (DIBINEM), University of Bologna, Bologna, Italy
You can also search for this author in PubMed Google Scholar
Contributions
BF drafted the work and substantively revised it; LL drafted the work; TP revised the work. All authors read and approved the final manuscript.
Corresponding author
Correspondence to Francesca Bisulli .
Ethics declarations
Ethics approval and consent to participate, consent for publication, competing interests.
The authors declare that they have no competing interests.
Additional information
Publisher’s note.
Springer Nature remains neutral with regard to jurisdictional claims in published maps and institutional affiliations.
Rights and permissions
Open Access This article is distributed under the terms of the Creative Commons Attribution 4.0 International License ( http://creativecommons.org/licenses/by/4.0/ ), which permits unrestricted use, distribution, and reproduction in any medium, provided you give appropriate credit to the original author(s) and the source, provide a link to the Creative Commons license, and indicate if changes were made. The Creative Commons Public Domain Dedication waiver ( http://creativecommons.org/publicdomain/zero/1.0/ ) applies to the data made available in this article, unless otherwise stated.
Reprints and permissions
About this article
Cite this article.
Bisulli, F., Licchetta, L. & Tinuper, P. Sleep related hyper motor epilepsy (SHE): a unique syndrome with heterogeneous genetic etiologies. Sleep Science Practice 3 , 3 (2019). https://doi.org/10.1186/s41606-019-0035-5
Download citation
Received : 25 March 2019
Accepted : 24 June 2019
Published : 17 July 2019
DOI : https://doi.org/10.1186/s41606-019-0035-5
Share this article
Anyone you share the following link with will be able to read this content:
Sorry, a shareable link is not currently available for this article.
Provided by the Springer Nature SharedIt content-sharing initiative
- Genetics; structural-genetic
Sleep Science and Practice
ISSN: 2398-2683
- General enquiries: [email protected]

- Cambridge Dictionary +Plus
Meaning of wandering in English
Your browser doesn't support HTML5 audio
wander verb ( MOVE AROUND )
- walk The baby has just learned to walk.
- stride She strode purposefully up to the desk and demanded to speak to the manager.
- march He marched right in to the office and demanded to see the governor.
- stroll We strolled along the beach.
- wander She wandered from room to room, not sure of what she was looking for.
- amble She ambled down the street, looking in shop windows.
- crawl There'd been a bad accident on the motorway and traffic was crawling.
- trundle Lorries trundle through the narrow lanes.
- creep He crept downstairs, hardly making any noise.
- trudge They trudged wearily through the snow.
- stroll We spent the afternoon strolling around Budapest.
- amble He ambled over to the window.
- Don't worry if you lose hold of the reins - the horse won't wander off.
- He wandered around, clearing up in a desultory way.
- We wandered along the shore , stepping over the flotsam that had washed up in the night .
- We wandered through the beautifully proportioned rooms of the Winter Palace.
- She was wandering around in a daze this morning .
- His eyes wandered over the posters adorning the walls .
- dumbwalking
You can also find related words, phrases, and synonyms in the topics:
wander verb ( SUBJECT )
- bury the lede idiom
- candy coating
- candy-coated
- circumlocution
- circumlocutory
- gloss over something
- go off on a tangent idiom
- mealy-mouthed
- prevaricate
- unexpressed
Related word
Examples of wandering.
In English, many past and present participles of verbs can be used as adjectives. Some of these examples may show the adjective use.
Word of the Day
to try to persuade a customer who is already buying something to buy more, or to buy something more expensive

Searching out and tracking down: talking about finding or discovering things

Learn more with +Plus
- Recent and Recommended {{#preferredDictionaries}} {{name}} {{/preferredDictionaries}}
- Definitions Clear explanations of natural written and spoken English English Learner’s Dictionary Essential British English Essential American English
- Grammar and thesaurus Usage explanations of natural written and spoken English Grammar Thesaurus
- Pronunciation British and American pronunciations with audio English Pronunciation
- English–Chinese (Simplified) Chinese (Simplified)–English
- English–Chinese (Traditional) Chinese (Traditional)–English
- English–Dutch Dutch–English
- English–French French–English
- English–German German–English
- English–Indonesian Indonesian–English
- English–Italian Italian–English
- English–Japanese Japanese–English
- English–Norwegian Norwegian–English
- English–Polish Polish–English
- English–Portuguese Portuguese–English
- English–Spanish Spanish–English
- English–Swedish Swedish–English
- Dictionary +Plus Word Lists
- wander (MOVE AROUND)
- wander (SUBJECT)
- All translations
To add wandering to a word list please sign up or log in.
Add wandering to one of your lists below, or create a new one.
{{message}}
Something went wrong.
There was a problem sending your report.
Five New Jersey towns named as best for night owls and nightlife

Five New Jersey towns are among the best destinations in the United States for those looking to enjoy the local nightlife, according to a recent survey.
Asbury Park (19th), Jersey City (48th), Hoboken (50th), Morristown (52nd) and Red Bank (72nd) all made the list compiled by photobook company Mixbook , which asked 3,000 experienced travelers to compile the top 150 areas for nocturnal activities.
"Whether it's lingering in 24-hour diners that serve breakfast at midnight, wandering through bustling night markets, or catching a midnight premiere at a local cinema, America's cities cater to the nocturnal soul seeking adventure, culture, and connection in the unique tranquility that only night can offer," the survey stated.
Asbury Park was recognized on the list for its "rich musical heritage," including the historic Stone Pony, and a variety of bars, restaurants and stores on the revitalized boardwalk. Jersey City and Hoboken, meanwhile, each boast plenty of late-night dining and drinking options along with picturesque views of the Manhattan skyline.
The other two New Jersey towns on the list made it in part for their respective music and entertainment theaters — the Mayo Performing Arts Center in Morristown and the Count Basie Center for the Arts in Red Bank. The survey specifically lauded Morristown's "vibrant nightlife with historic charm" as well as seasonal events like the Festival on the Green that bring in many visitors from out of town.
The top five nightlife areas on the Mixbook list are downtown Las Vegas; the French Quarter in New Orleans; Kailua-Kona, Hawaii; the Soho neighborhood of Manhattan; and downtown Anchorage, Alaska.

An official website of the United States government
The .gov means it’s official. Federal government websites often end in .gov or .mil. Before sharing sensitive information, make sure you’re on a federal government site.
The site is secure. The https:// ensures that you are connecting to the official website and that any information you provide is encrypted and transmitted securely.
- Publications
- Account settings
Preview improvements coming to the PMC website in October 2024. Learn More or Try it out now .
- Advanced Search
- Journal List
- Front Netw Physiol

The Mind After Midnight: Nocturnal Wakefulness, Behavioral Dysregulation, and Psychopathology
Andrew s. tubbs.
1 Sleep and Health Research Program, Department of Psychiatry, University of Arizona College of Medicine—Tucson, Tucson, AZ, United States
Fabian-Xosé Fernandez
2 Department of Psychology, Evelyn F Mcknight Brain Institute, University of Arizona, Tucson, AZ, United States
Michael A. Grandner
Michael l. perlis.
3 Department of Psychiatry, Perelman School of Medicine, University of Pennsylvania , Philadelphia, PA, United States
Elizabeth B. Klerman
4 Department of Neurology, Division of Sleep Medicine, Massachusetts General Hospital, Harvard Medical School, Boston, MA, United States
Sufficient sleep with minimal interruption during the circadian/biological night supports daytime cognition and emotional regulation. Conversely, disrupted sleep involving significant nocturnal wakefulness leads to cognitive and behavioral dysregulation. Most studies to-date have examined how fragmented or insufficient sleep affects next-day functioning, but recent work highlights changes in cognition and behavior that occur when someone is awake during the night. This review summarizes the evidence for day-night alterations in maladaptive behaviors, including suicide, violent crime, and substance use, and examines how mood, reward processing, and executive function differ during nocturnal wakefulness. Based on this evidence, we propose the Mind after Midnight hypothesis in which attentional biases, negative affect, altered reward processing, and prefrontal disinhibition interact to promote behavioral dysregulation and psychiatric disorders.
Introduction
Circadian rhythms influence human physiology and behavior, promoting wakefulness and cognition during the day and reducing cortical activity for sleep at night. Disrupted sleep increases the risk for incident and worsening psychiatric illness ( Pigeon et al., 2012 ; Li et al., 2016 ; Hertenstein et al., 2019 ; Zhang et al., 2019 ; Freeman et al., 2020 ), and this risk may partially derive from nocturnal wakefulness, which we define as being awake during the circadian or biological night. During the biological night, cognitive capacity and mood regulation are diminished and “reason sleeps” ( Perlis et al., 2016b ), probably due to both intrinsic sleep loss and circadian rhythm influences. This review examines the evidence for a nocturnal propensity for dysregulated behavior, explores neurophysiological changes that may facilitate such behavior, and presents the Mind After Midnight hypothesis that postulates that nocturnal wakefulness-associated changes in attentional biases, affective regulation, reward processing, and executive functioning set the stage for dysregulated behaviors and psychiatric disorders.
Nocturnal Changes in Dysregulated Behavior
Nighttime is associated with an increase in impulsive and maladaptive behaviors. The empirical data for four such behaviors are considered here: suicide/self-harm, violent crime, alcohol or other substance use, and food intake.
Suicide and Self-Harm
Although most suicides occur during the day, particularly from 10 AM to 2 PM ( Vollen and Watson, 1975 ; Barraclough, 1976 ; Williams and Tansella, 1987 ; Maldonado and Kraus, 1991 ; Altamura et al., 1999 ; Preti and Miotto, 2001 ; van Houwelingen and Beersma, 2001 ; Erazo et al., 2004 ), unadjusted counts are not appropriate to evaluate time-of-day differences in behavioral risk. Since wakefulness is a prerequisite to any volitional behavior, such analyses must also account for time-of-day changes in population wakefulness. In the United States, population wakefulness reaches its nadir around 3 AM, and then rises to maximal wakefulness between 10 AM and 8 PM. This means that the maximal incidence for any population-assessed behavior should be between 10 AM and 8 PM. Deviations from this pattern would reflect changes in underlying risk. For example, Perlis and colleagues ( Perlis et al., 2016a ) reported that the incident risk of suicide was three-fold greater between midnight and 6 AM than any other time of day ( Figure 1 ) after adjusting for population sleep/wake timing.

The prevalence and risk for suicide across 24 h. Although most suicides (grey bars, representing % of maximum hourly suicide count) occur around noon, population wakefulness (light grey shaded area) is also maximal at this time and throughout the afternoon and evening. After adjusting for population wakefulness, the incident risk ratio of suicide is substantially increased at night (black line, 95% confidence interval represented by dark grey band), with a 4.25-fold greater risk at 2AM than the 24-h average. Data are derived from the American Time Use Survey and the National Violent Death Reporting System for 2003 to 2010. Data are double-plotted to improve visualization of increased risk during nighttime hours.
Multiple subsequent analyses extend these initial findings. Veterans are eight-fold more likely to die by suicide between midnight and 3 AM ( McCarthy et al., 2019 ), and this nocturnal peak in suicide risk does not vary by month or method of suicide ( Tubbs et al., 2020b ). The nocturnal propensity for suicide is apparent in some populations without adjusting for population wakefulness : the raw suicide counts among Japanese males over the past 40 years peaked between midnight and 3 AM ( Boo et al., 2019 ), and the raw peak in suicides among heavy drinkers occurs around 9 PM compared to noon for non-heavy drinkers ( Chakravorty et al., 2018 ).
Nocturnal wakefulness may also increase risk for suicidal thinking. Objectively-measured wakefulness at 4 AM predicted next-day suicidal ideation in individuals with treatment-resistant depression ( Ballard et al., 2016 ), and self-reported wakefulness between 11 PM and 5 AM was associated with greater likelihood of suicidal ideation in both community ( Tubbs et al., 2020a ) and national samples ( Tubbs et al., 2021 ). A similar pattern emerges when examining data from the Internet forum Reddit: posts on a subforum called “SuicideWatch” peaked between 2 AM and 5 AM, whereas posts on a non-suicide related subforum called “AskReddit” peaked between 8 PM and 11 PM ( Dutta et al., 2021 ). The increase in volume of posts suggests that suicide-related thinking may be increased during those times, although the content of these posts was not analyzed.
Violent Behavior
Homicide and violent crime also increase in prevalence at night. A 1958 study reported that murders were more likely to occur between 6 PM and 1 AM ( Gibbens, 1958 ). Subsequent studies reported that murder rates in Manhattan peak between 6 PM and midnight ( Messner and Tardiff, 1985 ), and that homicides in Italy peak between midnight and 6 AM ( Sisti et al., 2012 ). More broadly, an estimated 55% of violent crimes are committed between 7 PM and 7 AM ( Kelsay et al., 2017 ), which seems only a minor difference except that most individuals are asleep during this interval. Thus, the relative incidence is likely higher at night than during the day. A review of attempted and completed rapes ( Belknap, 1987 ) showed that 39.5% of incidents occurred between 6 PM and midnight and 32% occurred between midnight and 6 AM. Thus, even without adjusting for population wakefulness, only 28.5% of rape incidents occur during the daytime.
Alcohol and Other Substance Use
Illicit or inappropriate use of substances increases during the night. A comparison of daytime-only versus 24-h operation of a supervised drug consumption center revealed a peak in substance use encounters at 10 PM and a 4.7-fold greater risk of opioid overdose at night, despite the reduced overall use of heroin at that time ( Montero-Moraga et al., 2020 ). Although circadian changes in metabolism may be responsible for this effect, this is, nonetheless, another example of how nocturnal wakefulness confers risk. Similarly, ecological momentary assessment studies have demonstrated that alcohol consumption among Latino youths is greater at night (63%) than any other time of day ( Comulada et al., 2016 ), and that alcohol cravings in adolescents peak at 8 PM and again at 2 AM ( Hisler et al., 2021 ). Later sleep timing in adolescents is also associated with at-risk alcohol use, binge drinking, and cannabis use ( Hasler et al., 2017 ), possibly due to consumption during the night when the ability to drink responsibly and/or in reasonable quantities may be impaired. Finally, awakening during the night is associated with relapse to cigarette smoking ( Boutou et al., 2008 ), perhaps due to an increased drive to smoke (withdrawal effect) and/or a reduced ability to not smoke (behavioral disinhibition).
Food Intake
Although prolonged wakefulness and insufficient sleep enhance metabolic demand with a concomitant increase in food intake, eating at night is not solely a consequence of metabolic demand. At night, food choices are skewed toward carbohydrates, lipids, and processed foods, and away from fruits and vegetables, with total intake often exceeding the caloric demands of prolonged wakefulness ( Knutson, 2012 ; Markwald et al., 2013 ; Shechter et al., 2014 ). These changes might reflect impairments in metabolic decision-making and/or an increased focus on hedonic reward ( Broussard and Van Cauter, 2016 ). One extreme example is people with night-eating syndrome, who consume the majority of their calories during uninhibited food intake at night ( Vander Wal, 2012 ). Although not well understood, night eating syndrome is hypothesized to involve changes in reward processing and serotonergic signaling ( Stunkard et al., 2009 ; Mendoza, 2018 ), all of which result from and contribute to inappropriate wakefulness at night. The possibility that nocturnal changes in reward circuitry motivate changes in food-related behavior is also seen in animal models; in mice, for example, homeostatic intake of food peaks during the nighttime active phase (the equivalent of the human day) while hedonic food intake peaks during the daytime rest phase (the equivalent of the human night) owing to changes in dopaminergic neurons ( Koch et al., 2020 ).
Nocturnal Changes in The Brain
Increased nocturnal risk for maladaptive behaviors likely results from sleep- and circadian-changes in neurophysiology during nocturnal wakefulness. Note that for this purpose, nocturnal wakefulness does not include short (e.g., <3 min) awakenings from sleep episodes. The following section discusses synaptic saturation, neurotransmitter activity, affect regulation, reward processing, and executive function as potential mechanisms of dysregulated nocturnal behaviors.
Synapses and Neurotransmitters
Under the synaptic homeostasis hypothesis ( Tononi and Cirelli, 2019 ), continuous wakefulness leads to synaptic saturation and impaired signal transmission. Much as a muscle fiber in continuous contraction loses strength, the saturated central nervous system eventually loses its capacity for effective cortical activity and cognition. Ordinarily, the sleep and circadian systems overlap at night to restore synaptic homeostasis via synaptic downscaling and reduced cortical excitability ( Ly et al., 2016 ). By contrast, wakefulness at night provokes cortical activity at a time when synapses are saturated and cortical responses are impaired.
Nocturnal wakefulness also involves circadian changes in neurotransmitter availability and signaling. Although norepinephrine and serotonin decrease at night in anticipation of sleep, dopamine peaks during the latter half of the dark period in both (diurnally active) humans ( Doran et al., 1990 ) and (nocturnally active) rats ( Castaneda et al., 2004 ), indicating it is tied to the rhythms of light and dark, not sleep and wake. This circadian rhythm in dopamine plays an important role in the regulation of sleep (particularly REM sleep) ( Monti and Monti, 2007 ; Kashiwagi et al., 2021 ), but may have unintended consequences when coincident with nocturnal wakefulness ( Alonso et al., 2021 ). Human studies show a reduction in available dopamine D2 receptors (inhibitory) in the ventral striatum following sleep deprivation ( Volkow et al., 2008 ; Volkow et al., 2012 ), which suggests an increase in dopaminergic activation. This may not only extend wakefulness but also adversely affect psychiatric symptoms associated with dopaminergic dysregulation (e.g., sensation seeking, impulsivity, delusional thinking). Nocturnal wakefulness may also produce a stress response with a surge in adrenergic signaling that further weakens prefrontal cortical activity, increases limbic/subcortical responsivity, and increases reflexive, impulsive decision-making ( Arnsten, 2015 ; Woo et al., 2021 ).
Positive and Negative Affect
Positive and negative affect exhibit distinct circadian rhythmicity. Positive affect peaks during the day and reaches its nadir during the night (1 AM to 4 AM) ( Murray et al., 2002 ; Murray et al., 2009 ; Golder and Macy, 2011 ; Hasler et al., 2012 ; Miller et al., 2015 ; Emens et al., 2020 ). Conversely, negative affect remains steady throughout the day before peaking sharply during the nighttime trough of positive affect ( Wirz-Justice, 2008 ; Kline et al., 2010 ; Golder and Macy, 2011 ; Dzogang et al., 2017 ; Emens et al., 2020 ; Ten Thij et al., 2020 ). Nocturnal wakefulness thus occurs at a time when positive affect is lowest and negative affect highest, which shapes how an individual attends to and interprets information. In-lab circadian investigations have shown that wakefulness during the habitual sleep period is linked to significantly worse subjective ratings of mood ( Boivin et al., 1997 ; Chellappa et al., 2020 ). Nocturnal wakefulness also impairs regulation of negative affect ( Harrington et al., 2021 ) and promotes depressive, anxious, and/or paranoid thinking ( Kahn-Greene et al., 2007 ; Killgore, 2010 ; Reeve et al., 2015 ; Sheaves et al., 2016 ; Cosgrave et al., 2018 ; Reeve et al., 2018 . Nocturnal wakefulness can also increase exposure to artificial light that can further disrupt sleep-wake rhythms and potentially tilt the balance of affect towards negative mood ( Bedrosian and Nelson, 2017 ).
Reward Processing
Reward is hypothesized to motivate behaviors that maximize survival. The survival benefit of many behaviors, however, varies as a function of time of day: for a diurnal animal, foraging for food may be beneficial during the day when food is visible, but dangerous at night due to predation. Preliminary evidence shows that activity in the nucleus accumbens, caudate, and putamen varies in accordance with the day/night cycle ( Byrne et al., 2019 ). In one study, the responses to high- and low-calorie foods in the putamen and ventral striatum were significantly reduced in the evening, even as subjective preoccupations about food were elevated ( Masterson et al., 2016 ). A comparison of striatal responses to a monetary reward task demonstrated that 1) reward activation was greatest in the afternoon ( Hasler et al., 2014 ), and 2) striatal activation during reward was significantly reduced during circadian misalignment ( Hasler et al., 2021 ). Thus, subcortical processing of reward appears to vary as a function of time of day.
Sleep deprivation adds another layer of complexity to reward processing as total sleep deprivation is known to increase risky decision-making ( Womack et al., 2013 ). Rewards following sleep loss are accompanied by greater activation of the ventral striatum ( Gujar et al., 2011 ) and reduced activity in the anterior insula ( Venkatraman et al., 2007 ; Venkatraman et al., 2011 ), possibly reflecting an exaggerated expectation of reward alongside diminished sensitivity to negative consequences. Interestingly, elevated activation in the ventral striatum at baseline predicts greater caloric and carbohydrate intake during the latter part of sleep deprivation ( Satterfield et al., 2018 ). This suggests that individuals with elevated baseline reward sensitivity are more likely to act impulsively (e.g., overeat) during sleep deprivation because of acute increases in activity in the nucleus accumbens. No studies to date have examined interactions between sleep deprivation and circadian timing on reward processing.
Executive Function
The prefrontal cortex integrates sensory input along with assessments of subcortical salience to manage adaptive behavioral responses related to long-term planning, risk-assessment, behavioral inhibition, and cognitive control (collectively referred to as executive function ( Diamond, 2013 )). Prefrontal performance is particularly sensitive to accumulating sleep debt as indexed by increased sleep delta activity (0.5–4.0 Hz on EEG), and enforced sleep deprivation results in maximal accumulation of delta activity over the prefrontal cortex relative to other brain areas ( Cajochen et al., 1999 ; Munch et al., 2004 ). Waking midline theta activity (4–8 Hz) arising from the anterior cingulate cortex also increases as a function of sustained wakefulness ( Cajochen et al., 1995 ; Cajochen et al., 1999 ; Finelli et al., 2000 ; Cavanagh and Frank, 2014 ). Even under protocols that include wakefulness during the biological night without sleep deprivation (i.e., forced desynchrony protocols), delta and waking theta frequency power in the EEG during sleep measured at night are greatest over the prefrontal region, which suggests that the rate at which wakefulness incurs sleep-pressure/cortical-fatigue is accelerated at night versus the day ( Cajochen et al., 1999 ; Finelli et al., 2000 ; Cajochen et al., 2002 ; Niggemyer et al., 2004 ; Munch et al., 2007 ). In addition, insufficient sleep and circadian changes associated with the biological night are linked to reduced cortical excitability ( Ly et al., 2016 ), reduced functional connectivity within the prefrontal cortex ( Verweij et al., 2014 ), and altered connectivity between the prefrontal cortex and the amygdala ( Killgore, 2013 ; Muto et al., 2016 ).
This frontal cortical dysfunction may explain the profound deficits in executive function observed during nocturnal wakefulness and/or following sleep deprivation ( Nilsson et al., 2005 ; Kuula et al., 2018 ; Cross et al., 2021 ). Cognitive functions such as working memory, complex attention, and problem solving deteriorate ( May and Kline, 1987 ; Libedinsky et al., 2013 ), leading to an increase in attentional lapses, performance errors, and injuries ( May and Kline, 1987 ; Nelson et al., 1995 ; Wright et al., 2012 ). Response inhibition is diminished ( Drummond et al., 2006 ), decision-making becomes inflexible ( Wimmer et al., 1992 ; Harrison and Horne, 1999 ; Maddox et al., 2009 ; Slama et al., 2018 ), and impaired risk management manifests in risky, net-loss decision-making ( Killgore et al., 2006 ; McKenna et al., 2007 ; Venkatraman et al., 2007 ; Pace-Schott et al., 2012 ; Womack et al., 2013 ; Mantua et al., 2021 ). Behavioral inhibition, which is maximized during the circadian day ( May and Hasher, 1998 ), declines as wakefulness proceeds into the circadian night ( Hasler et al., 2021 ). As an additional caveat, executive performance is significantly disrupted immediately upon awakening, a phenomenon known as sleep inertia. Cognitive deficits associated with sleep inertia are exacerbated by chronic short sleep and abrupt awakenings during the circadian night ( Horne and Moseley, 2011 ; McHill et al., 2019 ), suggesting that both sleep loss and circadian factors contribute to nocturnal deficits in executive function. Of note, some of these studies were performed in military, physician, or other participants who were highly skilled and motivated for the task ( May and Kline, 1987 ; Nelson et al., 1995 ; Harrison and Horne, 1999 ; Maddox et al., 2009 ; Horne and Moseley, 2011 ; Mantua et al., 2021 ). Unfortunately, in all these studies, assessment was done during the day, not during the night of sleep deprivation.
The Mind After Midnight
“Is it a bad thing to be awake when reason sleeps?” ( Perlis et al., 2016b ). Sleep loss- and circadian-dependent changes in molecular signaling, affect, reward processing, and executive function provide conceptual support for behavioral dysregulation, and empirical studies demonstrate a pattern of increased risk with nocturnal wakefulness. The reasons for being awake at night can also contribute to behavioral dysregulation: insomnia, nightmares, short sleep, or circadian rhythm disorders can produce nocturnal wakefulness, hypervigilance, and limited emotion regulation; hypnotics, alcohol, and other substances can produce rebound wakefulness, even if intoxication remains; and stress, anxiety, and mood disturbances promote nocturnal wakefulness even as they undermine decision-making. Figure 2 illustrates how biological and psychological factors intersect at nocturnal wakefulness and lead to The Mind After Midnight hypothesis, which we propose as a framework for interpreting current findings and a guide for future research and interventions, including therapy.

The Mind after Midnight. Blue boxes on the left and right sides indicate key processes within the model discussed in the text; grey boxes indicate additional components not specifically addressed. During nocturnal wakefulness, upregulation of the amygdala (AMY), nucleus accumbens (NAcc), and the rostral anterior cingulate cortex (rACC) increase emotionally driven salience, attention, and motivation, skew anticipation of risky rewards, and drive excess rumination. Conversely, impairments in the prefrontal cortex (PFC), dorsal anterior cingulate cortex (dACC) and anterior insula (AI) lead to executive dysfunction, diminished cognitive control, and insensitivity to error or loss. These changes promote a cycle of risky behaviors and consequences that can spiral out of control. Figure adapted from Perlis et al. (2016b) .
The Hypothesis
In brief, the circadian influence on (neuro)physiology differs over the 24 hours. During the day, molecular levels, neuronal activities and/or responsivity are tuned to our usual behavior, wake, which includes locomotor activity, eating, conscious interactions with our environment/people. During the night, these parameters are tuned to the usual behavior of sleep. So, if we are awake at these times, neurophysiology is prone to foster behavioral dysregulation, especially when these time-of-day effects are combined with sleep loss or disruption. We hypothesize that nocturnal wakefulness produces the Mind after Midnight, a combination of circadian-dependent decreases in molecular and cortical activity and responsiveness with sleep-loss-dependent alterations in synaptic signaling and cortical connectivity. Heightened negative affect and diminished positive affect produce a narrowed attentional focus on neutral or negative stimuli which are assigned incorrect or excessive emotional salience by an overactive amygdala. This biased information then feeds into an aberrant reward/motivation system characterized by elevated dopamine levels, altered dopamine receptor availability, increased activity in the nucleus accumbens (ventral striatum), and reduced activity in the caudate and putamen (dorsal striatum), which results in diminished activity during reward receipt but maximized anticipation/expectation of reward, particularly for risky behaviors.
This combination of negative attentional focus, skewed reward processing, and motivational impulsivity then rises to the prefrontal and anterior cingulate cortices. Ordinarily, coordinated activity between the dorsal anterior cingulate cortex (dACC), the rostral anterior cingulate cortex (rACC), and various regions of the prefrontal cortex (PFC) would exert cognitive control to suppress negative emotional distractors and focus on goal-oriented behavior ( Etkin et al., 2006 ; Buckner et al., 2008 ; Hamilton et al., 2011 ; Diener et al., 2012 ; Niendam et al., 2012 ; Shenhav et al., 2016 ; Berry et al., 2017 ). Sleep loss and nighttime circadian phase, however, are associated with synaptic saturation and cortical fatigue that disrupt this coordination and produce ruminative, self-referential thinking characterized by fear/anxiety, depression, and hopelessness ( Broyd et al., 2009 ; Sheline et al., 2009 ; Yoshimura et al., 2010 ; Toyoda et al., 2011 ; Nejad et al., 2013 ; Kaiser et al., 2015 ). Long-term planning and behavioral inhibition are diminished in favor of high-risk decision-making and cognitive inflexibility, leading to repeated, maladaptive behaviors that do not respond to negative feedback.
These conditions make it clear how poor mood, impaired judgement, and impulsivity could lead to maladaptive behaviors and catastrophic outcomes. For example, a previously abstinent heroin user who successfully manages cravings during the day may experience greater cravings and diminished resistance at night. The appeal of heroin use becomes more desirable and satisfying than the potential costs, and a single impulsive decision leads to a relapse. Further, a dose that was sufficiently rewarding during the day may be insufficient at night, potentially resulting in an increased dose, repeated use, or both, as the risk of overdose is downplayed or discarded. If this process repeats night after night, a conditioned pattern of nighttime heroin use may emerge ( Gillman et al., 2019 ).
Another example is a college student experiencing nocturnal wakefulness due to a delayed sleep schedule and insomnia. Negative mood is at its peak, and the student feels isolated and alone, leading to endless rumination on prior negative relationship experiences. These particular experiences quickly, and inappropriately, generalize to all relationships, thus creating a sense of hopelessness and despair. Suicide, previously inconceivable, emerges as an escape from loneliness and pain, and before the costs of suicide are considered the student has acquired the means and is prepared to act at a time when no one is awake to stop them.
These examples demonstrate how negative affect, impaired judgment, and impulsivity during nocturnal wakefulness may increase the incidence and appeal of dangerous ideas, and promote dysregulated behaviors.
Future Directions
The Mind after Midnight hypothesis conceptualizes how disrupted or decreased sleep could contribute to psychiatric disorders and unsafe behaviors, and offers a unique opportunity for meaningful intervention. The Mind after Midnight hypothesis also integrates well with psychological models of acute behavioral risk, such as the Suicide Crisis Syndrome or Acute Suicidal Affective Disturbance ( Schuck et al., 2019 ; Voros et al., 2021 ).
All aspects of this hypothesis require empirical validation. We have reviewed reports of data collected during the biological night, and, when such data were not available, we used reports of data from after a night of sleep loss. To test the Mind after Midnight hypothesis, data need to be collected during the biological night (including - when possible - using protocols that do not cause sleep loss). Adding time-of-day as a relevant metric to all studies is also extremely important, as measuring time-of-day (and time since awakening) effects on a variety of outcomes could identify behaviors (or individuals) that are most vulnerable or resilient to nocturnal wakefulness. Mechanistic studies could use known electrophysiological measures of cognition, emotion, and cortical activity (e.g., frontal alpha asymmetry, frontal midline theta, fMRI) to measure nocturnal trait and state changes in activity. Future work could also distinguish the effects of prolonged wakefulness from abrupt nighttime awakenings and the distinctions between sleep loss and circadian processes.
The Mind after Midnight naturally lends itself to clinical applications. The simplest solution would be to help vulnerable individuals to sleep through the night, thus reducing their exposure to the times with elevated risk due to behavioral dysregulation. Example interventions would include treatment for insomnia, pain, cravings, or other causes of nighttime awakening, changes in the environment, and/or the provision of greater social supports when individuals are awake at night.
Funding Statement
AT: DOD W81XWH-16-2-0003 (F-XF, MG) NIH K24AG055602 (MP). F-XF: Velux Stiftung (Project No. 1360). MP: NIH K24AG055602, R01AG054521. MG: R01DA051321 and R01MD011600. EK: NIH K24-HL105664, U54-AG062322, R21DA052861.
Author Contributions
AT, MP, MG, and EK conceived the original concepts and framework. F-XF provided subsequent input on the ideas and arguments. AT drafted the manuscript and figures, which were subsequently revised by F-XF, MP, MG, and EK. All authors reviewed and approved the final version of the manuscript.
Conflict of Interest
MG received grants from Jazz Pharmaceuticals and CeraZ; served as a paid consultant to Idorsia, Fitbit, Natrol, Smartypants Vitamins, Athleta, Casper Sleep, and Lyma; and serves as an advisor to Nightfood and Simple Habit. EK is consulting for Circadian Therapeutics, The National Sleep Foundation, Sanofi-Genzyme. Partner owns Chronsulting.
The remaining authors declare that the research was conducted in the absence of any commercial or financial relationships that could be construed as a potential conflict of interest.
Publisher’s Note
All claims expressed in this article are solely those of the authors and do not necessarily represent those of their affiliated organizations, or those of the publisher, the editors and the reviewers. Any product that may be evaluated in this article, or claim that may be made by its manufacturer, is not guaranteed or endorsed by the publisher.
- Alonso I. P., Pino J. A., Kortagere S., Torres G. E., España R. A. (2021). Dopamine Transporter Function Fluctuates across Sleep/wake State: Potential Impact for Addiction . Neuropsychopharmacol. 46 ( 4 ), 699–708. 10.1038/s41386-020-00879-2 [ PMC free article ] [ PubMed ] [ CrossRef ] [ Google Scholar ]
- Altamura C., VanGastel A., Pioli R., Mannu P., Michael Maes M. (1999). Seasonal and Circadian Rhythms in Suicide in Cagliari, Italy . J. Affective Disord. 53 ( 1 ), 77–85. 10.1016/s0165-0327(98)00099-8 10.1016/s0165-0327(98)00099-8 [ PubMed ] [ CrossRef ] [ CrossRef ] [ Google Scholar ]
- Arnsten A. F. T. (2015). Stress Weakens Prefrontal Networks: Molecular Insults to Higher Cognition . Nat. Neurosci. 18 ( 10 ), 1376–1385. 10.1038/nn.4087 [ PMC free article ] [ PubMed ] [ CrossRef ] [ Google Scholar ]
- Ballard E. D., Vande Voort J. L., Bernert R. A., Luckenbaugh D. A., Richards E. M., Niciu M. J., et al. (2016). Nocturnal Wakefulness Is Associated with Next-Day Suicidal Ideation in Major Depressive Disorder and Bipolar Disorder . J. Clin. Psychiatry 77 ( 6 ), 825–831. 10.4088/JCP.15m09943 [ PMC free article ] [ PubMed ] [ CrossRef ] [ Google Scholar ]
- Barraclough B. M. (1976). Time of Day Chosen for Suicide . Psychol. Med. 6 ( 2 ), 303–305. 10.1017/s0033291700013866 [ PubMed ] [ CrossRef ] [ Google Scholar ]
- Bedrosian T. A., Nelson R. J. (2017). Timing of Light Exposure Affects Mood and Brain Circuits . Transl Psychiatry 7 ( 1 ), e1017. 10.1038/tp.2016.262 [ PMC free article ] [ PubMed ] [ CrossRef ] [ Google Scholar ]
- Belknap J. (1987). Routine Activity Theory and the Risk of Rape: Analyzing Ten Years of National Crime Survey Data . Criminal Justice Pol. Rev. 2 ( 4 ), 337–356. 10.1177/088740348700200403 [ CrossRef ] [ Google Scholar ]
- Berry A. S., Sarter M., Lustig C. (2017). Distinct Frontoparietal Networks Underlying Attentional Effort and Cognitive Control . J. Cogn. Neurosci. 29 ( 7 ), 1212–1225. 10.1162/jocn_a_01112 [ PMC free article ] [ PubMed ] [ CrossRef ] [ Google Scholar ]
- Boivin D. B., Czeisler C. A., Dijk D. J., Duffy J. F., Folkard S., Minors D. S., et al. (1997). Complex Interaction of the Sleep-Wake Cycle and Circadian Phase Modulates Mood in Healthy Subjects . Arch. Gen. Psychiatry 54 ( 2 ), 145–152. 10.1001/archpsyc.1997.01830140055010 [ PubMed ] [ CrossRef ] [ Google Scholar ]
- Boo J., Matsubayashi T., Ueda M. (2019). Diurnal Variation in Suicide Timing by Age and Gender: Evidence from Japan across 41 Years . J. Affective Disord. 243 , 366–374. 10.1016/j.jad.2018.09.030 [ PubMed ] [ CrossRef ] [ Google Scholar ]
- Boutou A. K., Tsiata E. A., Pataka A., Kontou P. K., Pitsiou G. G., Argyropoulou P. (2008). Smoking Cessation in Clinical Practice: Predictors of Six-Month Continuous Abstinence in a Sample of Greek Smokers . Prim. Care Respir. J. 17 ( 1 ), 32–38. 10.3132/pcrj.2008.00009 [ PMC free article ] [ PubMed ] [ CrossRef ] [ Google Scholar ]
- Broussard J. L., Van Cauter E. (2016). Disturbances of Sleep and Circadian Rhythms . Curr. Opin. Endocrinol. Diabetes Obes. 23 ( 5 ), 353–359. 10.1097/MED.0000000000000276 [ PMC free article ] [ PubMed ] [ CrossRef ] [ Google Scholar ]
- Broyd S. J., Demanuele C., Debener S., Helps S. K., James C. J., Sonuga-Barke E. J. S. (2009). Default-mode Brain Dysfunction in Mental Disorders: a Systematic Review . Neurosci. Biobehavioral Rev. 33 ( 3 ), 279–296. 10.1016/j.neubiorev.2008.09.002 [ PubMed ] [ CrossRef ] [ Google Scholar ]
- Buckner R. L., Andrews-Hanna J. R., Schacter D. L. (2008). The Brain's Default Network . Ann. N. Y Acad. Sci. 1124 , 1–38. 10.1196/annals.1440.011 [ PubMed ] [ CrossRef ] [ Google Scholar ]
- Byrne J. E. M., Tremain H., Leitan N. D., Keating C., Johnson S. L., Murray G. (2019). Circadian Modulation of Human Reward Function: Is There an Evidentiary Signal in Existing Neuroimaging Studies . Neurosci. Biobehavioral Rev. 99 , 251–274. 10.1016/j.neubiorev.2019.01.025 [ PubMed ] [ CrossRef ] [ Google Scholar ]
- Cajochen C., Foy R., Dijk D. J. (1999). Frontal Predominance of a Relative Increase in Sleep delta and Theta EEG Activity after Sleep Loss in Humans . Sleep Res. Online 2 ( 3 ), 65–69. [ PubMed ] [ Google Scholar ]
- Cajochen C., Brunner D. P., Krauchi K., Graw P., Wirz-Justice A. (1995). Power Density in Theta/alpha Frequencies of the Waking EEG Progressively Increases during Sustained Wakefulness . Sleep 18 ( 10 ), 890–894. 10.1093/sleep/18.10.890 [ PubMed ] [ CrossRef ] [ Google Scholar ]
- Cajochen C., Wyatt J. K., Czeisler C. A., Dijk D. J. (2002). Separation of Circadian and Wake Duration-dependent Modulation of EEG Activation during Wakefulness . Neuroscience 114 ( 4 ), 1047–1060. 10.1016/s0306-4522(02)00209-9 10.1016/s0306-4522(02)00209-9 [ PubMed ] [ CrossRef ] [ CrossRef ] [ Google Scholar ]
- Castañeda T. R., Prado B. M., Prieto D., Mora F. (2004). Circadian Rhythms of Dopamine, Glutamate and GABA in the Striatum and Nucleus Accumbens of the Awake Rat: Modulation by Light . J. Pineal Res. 36 ( 3 ), 177–185. 10.1046/j.1600-079x.2003.00114.x [ PubMed ] [ CrossRef ] [ Google Scholar ]
- Cavanagh J. F., Frank M. J. (2014). Frontal Theta as a Mechanism for Cognitive Control . Trends Cogn. Sci. 18 ( 8 ), 414–421. 10.1016/j.tics.2014.04.012 [ PMC free article ] [ PubMed ] [ CrossRef ] [ Google Scholar ]
- Chakravorty S., Smith R. V., Perlis M. L., Grandner M. A., Kranzler H. R. (2018). Circadian Pattern of Deaths Due to Suicide in Intoxicated Alcohol-dependent Individuals . J. Clin. Psychiatry 79 ( 6 ), 17m11800. 10.4088/JCP.17m11800 [ PMC free article ] [ PubMed ] [ CrossRef ] [ Google Scholar ]
- Chellappa S. L., Morris C. J., Scheer F. A. J. L. (2020). Circadian Misalignment Increases Mood Vulnerability in Simulated Shift Work . Sci. Rep. 10 ( 1 ), 18614. 10.1038/s41598-020-75245-9 [ PMC free article ] [ PubMed ] [ CrossRef ] [ Google Scholar ]
- Comulada W. S., Swendeman D., Wu N. (2016). Cell Phone-Based Ecological Momentary Assessment of Substance Use Context for Latino Youth in Outpatient Treatment: Who, what, when and where . Drug and Alcohol Dependence 167 , 207–213. 10.1016/j.drugalcdep.2016.08.623 [ PMC free article ] [ PubMed ] [ CrossRef ] [ Google Scholar ]
- Cosgrave J., Haines R., van Heugten-van der Kloet D., Purple R., Porcheret K., Foster R., et al. (2018). The Interaction between Subclinical Psychotic Experiences, Insomnia and Objective Measures of Sleep . Schizophrenia Res. 193 , 204–208. 10.1016/j.schres.2017.06.058 [ PMC free article ] [ PubMed ] [ CrossRef ] [ Google Scholar ]
- Cross N. E., Pomares F. B., Nguyen A., Perrault A. A., Jegou A., Uji M., et al. (2021). An Altered Balance of Integrated and Segregated Brain Activity Is a Marker of Cognitive Deficits Following Sleep Deprivation . Plos Biol. 19 ( 11 ), e3001232. 10.1371/journal.pbio.3001232 [ PMC free article ] [ PubMed ] [ CrossRef ] [ Google Scholar ]
- Diamond A. (2013). Executive Functions . Annu. Rev. Psychol. 64 , 135–168. 10.1146/annurev-psych-113011-143750 [ PMC free article ] [ PubMed ] [ CrossRef ] [ Google Scholar ]
- Diener C., Kuehner C., Brusniak W., Ubl B., Wessa M., Flor H. (2012). A Meta-Analysis of Neurofunctional Imaging Studies of Emotion and Cognition in Major Depression . Neuroimage 61 ( 3 ), 677–685. 10.1016/j.neuroimage.2012.04.005 [ PubMed ] [ CrossRef ] [ Google Scholar ]
- Doran A. R., Labarca R., Wolkowitz O. M., Roy A., Douillet P., Pickar D. (1990). Circadian Variation of Plasma Homovanillic Acid Levels Is Attentuated by Fluphenazine in Patients with Schizophrenia . Arch. Gen. Psychiatry 47 ( 6 ), 558–563. 10.1001/archpsyc.1990.01810180058009 [ PubMed ] [ CrossRef ] [ Google Scholar ]
- Drummond S. P. A., Paulus M. P., Tapert S. F. (2006). Effects of Two Nights Sleep Deprivation and Two Nights Recovery Sleep on Response Inhibition . J. Sleep Res. 15 ( 3 ), 261–265. 10.1111/j.1365-2869.2006.00535.x [ PubMed ] [ CrossRef ] [ Google Scholar ]
- Dutta R., Gkotsis G., Velupillai S., Bakolis I., Stewart R. (2021). Temporal and Diurnal Variation in Social media Posts to a Suicide Support Forum . BMC Psychiatry 21 ( 1 ), 259. 10.1186/s12888-021-03268-1 [ PMC free article ] [ PubMed ] [ CrossRef ] [ Google Scholar ]
- Dzogang F., Lightman S., Cristianini N. (2017). Circadian Mood Variations in Twitter Content . Brain Neurosci. Adv. 1 , 239821281774450. 10.1177/2398212817744501 [ PMC free article ] [ PubMed ] [ CrossRef ] [ Google Scholar ]
- Emens J. S., Berman A. M., Thosar S. S., Butler M. P., Roberts S. A., Clemons N. A., et al. (2020). Circadian Rhythm in Negative Affect: Implications for Mood Disorders . Psychiatry Res. 293 , 113337. 10.1016/j.psychres.2020.113337 [ PMC free article ] [ PubMed ] [ CrossRef ] [ Google Scholar ]
- Erazo N., Baumert J., Ladwig K.-H. (2004). Sex-specific Time Patterns of Suicidal Acts on the German Railway System. An Analysis of 4003 Cases . J. Affective Disord. 83 ( 1 ), 1–9. 10.1016/j.jad.2004.04.012 [ PubMed ] [ CrossRef ] [ Google Scholar ]
- Etkin A., Egner T., Peraza D. M., Kandel E. R., Hirsch J. (2006). Resolving Emotional Conflict: a Role for the Rostral Anterior Cingulate Cortex in Modulating Activity in the Amygdala . Neuron 51 ( 6 ), 871–882. 10.1016/j.neuron.2006.07.029 [ PubMed ] [ CrossRef ] [ Google Scholar ]
- Finelli L. A., Baumann H., Borbély A. A., Achermann P. (2000). Dual Electroencephalogram Markers of Human Sleep Homeostasis: Correlation between Theta Activity in Waking and Slow-Wave Activity in Sleep . Neuroscience 101 ( 3 ), 523–529. 10.1016/s0306-4522(00)00409-7 10.1016/s0306-4522(00)00409-7 [ PubMed ] [ CrossRef ] [ CrossRef ] [ Google Scholar ]
- Freeman D., Sheaves B., Waite F., Harvey A. G., Harrison P. J. (2020). Sleep Disturbance and Psychiatric Disorders . The Lancet Psychiatry 7 ( 7 ), 628–637. 10.1016/S2215-0366(20)30136-X 10.1016/s2215-0366(20)30136-x [ PubMed ] [ CrossRef ] [ CrossRef ] [ Google Scholar ]
- Gibbens T. C. N. (1958). Sane and Insane Homicide . J. Criminal L. Criminology, Police Sci. 49 ( 2 ), 110–115. 10.2307/1140920 [ CrossRef ] [ Google Scholar ]
- Gillman A. G., Rebec G. V., Pecoraro N. C., Kosobud A. E. K. (2019). Circadian Entrainment by Food and Drugs of Abuse . Behav. Process. 165 , 23–28. 10.1016/j.beproc.2019.05.017 [ PMC free article ] [ PubMed ] [ CrossRef ] [ Google Scholar ]
- Golder S. A., Macy M. W. (2011). Diurnal and Seasonal Mood Vary with Work, Sleep, and Daylength across Diverse Cultures . Science 333 ( 6051 ), 1878–1881. 10.1126/science.1202775 [ PubMed ] [ CrossRef ] [ Google Scholar ]
- Gujar N., Yoo S.-S., Hu P., Walker M. P. (2011). Sleep Deprivation Amplifies Reactivity of Brain Reward Networks, Biasing the Appraisal of Positive Emotional Experiences . J. Neurosci. 31 ( 12 ), 4466–4474. 10.1523/JNEUROSCI.3220-10.2011 [ PMC free article ] [ PubMed ] [ CrossRef ] [ Google Scholar ]
- Hamilton J. P., Furman D. J., Chang C., Thomason M. E., Dennis E., Gotlib I. H. (2011). Default-mode and Task-Positive Network Activity in Major Depressive Disorder: Implications for Adaptive and Maladaptive Rumination . Biol. Psychiatry 70 ( 4 ), 327–333. 10.1016/j.biopsych.2011.02.003 [ PMC free article ] [ PubMed ] [ CrossRef ] [ Google Scholar ]
- Harrington M. O., Ashton J. E., Sankarasubramanian S., Anderson M. C., Cairney S. A. (2021). Losing Control: Sleep Deprivation Impairs the Suppression of Unwanted Thoughts . Clin. Psychol. Sci. 9 ( 1 ), 97–113. 10.1177/2167702620951511 [ PMC free article ] [ PubMed ] [ CrossRef ] [ Google Scholar ]
- Harrison Y., Horne J. A. (1999). One Night of Sleep Loss Impairs Innovative Thinking and Flexible Decision Making . Organizational Behav. Hum. Decis. Process. 78 ( 2 ), 128–145. 10.1006/obhd.1999.2827 [ PubMed ] [ CrossRef ] [ Google Scholar ]
- Hasler B. P., Forbes E. E., Franzen P. L. (2014). Time-of-day Differences and Short-Term Stability of the Neural Response to Monetary Reward: a Pilot Study . Psychiatry Res. Neuroimaging 224 ( 1 ), 22–27. 10.1016/j.pscychresns.2014.07.005 [ PMC free article ] [ PubMed ] [ CrossRef ] [ Google Scholar ]
- Hasler B. P., Franzen P. L., de Zambotti M., Prouty D., Brown S. A., Tapert S. F., et al. (2017). Eveningness and Later Sleep Timing Are Associated with Greater Risk for Alcohol and Marijuana Use in Adolescence: Initial Findings from the National Consortium on Alcohol and Neurodevelopment in Adolescence Study . Alcohol. Clin. Exp. Res. 41 ( 6 ), 1154–1165. 10.1111/acer.13401 [ PMC free article ] [ PubMed ] [ CrossRef ] [ Google Scholar ]
- Hasler B. P., Germain A., Nofzinger E. A., Kupfer D. J., Krafty R. T., Rothenberger S. D., et al. (2012). Chronotype and Diurnal Patterns of Positive Affect and Affective Neural Circuitry in Primary Insomnia . J. Sleep Res. 21 ( 5 ), 515–526. 10.1111/j.1365-2869.2012.01002.x [ PMC free article ] [ PubMed ] [ CrossRef ] [ Google Scholar ]
- Hasler B. P., Soehner A. M., Wallace M. L., Logan R. W., Ngari W., Forbes E. E., et al. (2021). Experimentally Imposed Circadian Misalignment Alters the Neural Response to Monetary Rewards and Response Inhibition in Healthy Adolescents . Psychol. Med. 17 , 1–9. 10.1017/S0033291721000787 [ PMC free article ] [ PubMed ] [ CrossRef ] [ Google Scholar ]
- Hertenstein E., Feige B., Gmeiner T., Kienzler C., Spiegelhalder K., Johann A., et al. (2019). Insomnia as a Predictor of Mental Disorders: A Systematic Review and Meta-Analysis . Sleep Med. Rev. 43 , 96–105. 10.1016/j.smrv.2018.10.006 [ PubMed ] [ CrossRef ] [ Google Scholar ]
- Hisler G. C., Rothenberger S. D., Clark D. B., Hasler B. P. (2021). Is There a 24-hour Rhythm in Alcohol Craving and Does it Vary by Sleep/circadian Timing . Chronobiology Int. 38 ( 1 ), 109–121. 10.1080/07420528.2020.1838532 [ PMC free article ] [ PubMed ] [ CrossRef ] [ Google Scholar ]
- Horne J., Moseley R. (2011). Sudden Early-Morning Awakening Impairs Immediate Tactical Planning in a Changing 'emergency' Scenario . J. Sleep Res. 20 ( 2 ), 275–278. 10.1111/j.1365-2869.2010.00904.x [ PubMed ] [ CrossRef ] [ Google Scholar ]
- Kahn-Greene E. T., Killgore D. B., Kamimori G. H., Balkin T. J., Killgore W. D. S. (2007). The Effects of Sleep Deprivation on Symptoms of Psychopathology in Healthy Adults . Sleep Med. 8 ( 3 ), 215–221. 10.1016/j.sleep.2006.08.007 [ PubMed ] [ CrossRef ] [ Google Scholar ]
- Kaiser R. H., Andrews-Hanna J. R., Wager T. D., Pizzagalli D. A. (2015). Large-Scale Network Dysfunction in Major Depressive Disorder . JAMA Psychiatry 72 ( 6 ), 603–611. 10.1001/jamapsychiatry.2015.0071 [ PMC free article ] [ PubMed ] [ CrossRef ] [ Google Scholar ]
- Kashiwagi M., Kanuka M., Tanaka K., Fujita M., Nakai A., Tatsuzawa C., et al. (2021). Impaired Wakefulness and Rapid Eye Movement Sleep in Dopamine-Deficient Mice . Mol. Brain 14 ( 1 ), 170. 10.1186/s13041-021-00879-3 [ PMC free article ] [ PubMed ] [ CrossRef ] [ Google Scholar ]
- Kelsay J. D., Tillyer M. S., Tillyer R., Ward J. T. (2017). The Violent Victimization of Children, Adolescents, Adults, and the Elderly: Situational Characteristics and Victim Injury . Violence Vict 32 ( 2 ), 342–361. 10.1891/0886-6708.VV-D-16-00004 [ PubMed ] [ CrossRef ] [ Google Scholar ]
- Killgore W. D. S., Balkin T. J., Wesensten N. J. (2006). Impaired Decision Making Following 49 H of Sleep Deprivation . J. Sleep Res. 15 ( 1 ), 7–13. 10.1111/j.1365-2869.2006.00487.x [ PubMed ] [ CrossRef ] [ Google Scholar ]
- Killgore W. D. S. (2010). Effects of Sleep Deprivation on Cognition . Prog. Brain Res. 185 , 105–129. 10.1016/B978-0-444-53702-7.00007-5 [ PubMed ] [ CrossRef ] [ Google Scholar ]
- Killgore W. D. S. (2013). Self-reported Sleep Correlates with Prefrontal-Amygdala Functional Connectivity and Emotional Functioning . Sleep 36 ( 11 ), 1597–1608. 10.5665/sleep.3106 [ PMC free article ] [ PubMed ] [ CrossRef ] [ Google Scholar ]
- Kline C. E., Durstine J. L., Davis J. M., Moore T. A., Devlin T. M., Youngstedt S. D. (2010). Circadian Rhythms of Psychomotor Vigilance, Mood, and Sleepiness in the Ultra-short Sleep/wake Protocol . Chronobiology Int. 27 ( 1 ), 161–180. 10.3109/07420521003648604 [ PMC free article ] [ PubMed ] [ CrossRef ] [ Google Scholar ]
- Knutson K. L. (2012). Does Inadequate Sleep Play a Role in Vulnerability to Obesity . Am. J. Hum. Biol. 24 ( 3 ), 361–371. 10.1002/ajhb.22219 [ PMC free article ] [ PubMed ] [ CrossRef ] [ Google Scholar ]
- Koch C. E., Begemann K., Kiehn J. T., Griewahn L., Mauer J., Hess M. E., et al. (2020). Circadian Regulation of Hedonic Appetite in Mice by Clocks in Dopaminergic Neurons of the VTA . Nat. Commun. 11 ( 1 ), 3071. 10.1038/s41467-020-16882-6 [ PMC free article ] [ PubMed ] [ CrossRef ] [ Google Scholar ]
- Kuula L., Pesonen A.-K., Heinonen K., Kajantie E., Eriksson J. G., Andersson S., et al. (2018). Naturally Occurring Circadian Rhythm and Sleep Duration Are Related to Executive Functions in Early Adulthood . J. Sleep Res. 27 ( 1 ), 113–119. 10.1111/jsr.12581 [ PubMed ] [ CrossRef ] [ Google Scholar ]
- Li L., Wu C., Gan Y., Qu X., Lu Z. (2016). Insomnia and the Risk of Depression: a Meta-Analysis of Prospective Cohort Studies . BMC Psychiatry 16 ( 1 ), 375. 10.1186/s12888-016-1075-3 [ PMC free article ] [ PubMed ] [ CrossRef ] [ Google Scholar ]
- Libedinsky C., Massar S. A. A., Ling A., Chee W., Huettel S. A., Chee M. W. L. (2013). Sleep Deprivation Alters Effort Discounting but Not Delay Discounting of Monetary Rewards . Sleep 36 ( 6 ), 899–904. 10.5665/sleep.2720 [ PMC free article ] [ PubMed ] [ CrossRef ] [ Google Scholar ]
- Ly J. Q. M., Gaggioni G., Chellappa S. L., Papachilleos S., Brzozowski A., Borsu C., et al. (2016). Circadian Regulation of Human Cortical Excitability . Nat. Commun. 7 , 11828. 10.1038/ncomms11828 [ PMC free article ] [ PubMed ] [ CrossRef ] [ Google Scholar ]
- Maddox W. T., Glass B. D., Wolosin S. M., Savarie Z. R., Bowen C., Matthews M. D., et al. (2009). The Effects of Sleep Deprivation on Information-Integration Categorization Performance . Sleep 32 ( 11 ), 1439–1448. 10.1093/sleep/32.11.1439 [ PMC free article ] [ PubMed ] [ CrossRef ] [ Google Scholar ]
- Maldonado G., Kraus J. F. (1991). Variation in Suicide Occurrence by Time of Day, Day of the Week, Month, and Lunar Phase . Suicide Life Threat Behav. 21 ( 2 ), 174–187. [ PubMed ] [ Google Scholar ]
- Mantua J., Bessey A. F., Mickelson C. A., Choynowski J. J., Noble J. J., Burke T. M., et al. (2021). Sleep and High-Risk Behavior in Military Service Members: a Mega-Analysis of Four Diverse U.S. Army Units . Sleep 44 ( 4 ), zsaa221. 10.1093/sleep/zsaa221 [ PubMed ] [ CrossRef ] [ Google Scholar ]
- Markwald R. R., Melanson E. L., Smith M. R., Higgins J., Perreault L., Eckel R. H., et al. (2013). Impact of Insufficient Sleep on Total Daily Energy Expenditure, Food Intake, and Weight Gain . Proc. Natl. Acad. Sci. 110 ( 14 ), 5695–5700. 10.1073/pnas.1216951110 [ PMC free article ] [ PubMed ] [ CrossRef ] [ Google Scholar ]
- Masterson T. D., Kirwan C. B., Davidson L. E., LeCheminant J. D. (2016). Neural Reactivity to Visual Food Stimuli Is Reduced in Some Areas of the Brain during Evening Hours Compared to Morning Hours: an fMRI Study in Women . Brain Imaging Behav. 10 ( 1 ), 68–78. 10.1007/s11682-015-9366-8 [ PubMed ] [ CrossRef ] [ Google Scholar ]
- May C. P., Hasher L. (1998). Synchrony Effects in Inhibitory Control over Thought and Action . J. Exp. Psychol. Hum. Perception Perform. 24 ( 2 ), 363–379. 10.1037//0096-1523.24.2.36310.1037/0096-1523.24.2.363 [ PubMed ] [ CrossRef ] [ Google Scholar ]
- May J., Kline P. (1987). Measuring the Effects upon Cognitive Abilities of Sleep Loss during Continuous Operations . Br. J. Psychol. 78 ( Pt 4 ), 443–455. 10.1111/j.2044-8295.1987.tb02261.x [ PubMed ] [ CrossRef ] [ Google Scholar ]
- McCarthy M. S., Hoffmire C., Brenner L. A., Nazem S. (2019). Sleep and Timing of Death by Suicide Among U.S. Veterans 2006-2015: Analysis of the American Time Use Survey and the National Violent Death Reporting System . Sleep 42 ( 8 ), zsz094. 10.1093/sleep/zsz094 [ PubMed ] [ CrossRef ] [ Google Scholar ]
- McHill A. W., Hull J. T., Cohen D. A., Wang W., Czeisler C. A., Klerman E. B. (2019). Chronic Sleep Restriction Greatly Magnifies Performance Decrements Immediately after Awakening . Sleep 42 ( 5 ), zsz032. 10.1093/sleep/zsz032 [ PMC free article ] [ PubMed ] [ CrossRef ] [ Google Scholar ]
- McKenna B. S., Dickinson D. L., Orff H. J., Drummond S. P. A. (2007). The Effects of One Night of Sleep Deprivation on Known-Risk and Ambiguous-Risk Decisions . J. Sleep Res. 16 ( 3 ), 245–252. 10.1111/j.1365-2869.2007.00591.x [ PubMed ] [ CrossRef ] [ Google Scholar ]
- Mendoza J. (2019). Food Intake and Addictive-like Eating Behaviors: Time to Think about the Circadian Clock(s) . Neurosci. Biobehavioral Rev. 106 , 122–132. 10.1016/j.neubiorev.2018.07.003 [ PubMed ] [ CrossRef ] [ Google Scholar ]
- Messner S. F., Tardiff K. (1985). The Social Ecology of Urban Homicide: An Application of the “Routine Activities” Approach . Criminology 23 ( 2 ), 241–267. 10.1111/j.1745-9125.1985.tb00336.x [ CrossRef ] [ Google Scholar ]
- Miller M. A., Rothenberger S. D., Hasler B. P., Donofry S. D., Wong P. M., Manuck S. B., et al. (2015). Chronotype Predicts Positive Affect Rhythms Measured by Ecological Momentary Assessment . Chronobiology Int. 32 ( 3 ), 376–384. 10.3109/07420528.2014.983602 [ PMC free article ] [ PubMed ] [ CrossRef ] [ Google Scholar ]
- Montero-Moraga J. M., Garrido-Albaina A., Barbaglia M. G., Gotsens M., Aranega D., Espelt A., et al. (2020). Impact of 24-hour Schedule of a Drug Consumption Room on Service Use and Number of Non-fatal Overdoses. A Quasiexperimental Study in Barcelona . Int. J. Drug Pol. 81 , 102772. 10.1016/j.drugpo.2020.102772 [ PubMed ] [ CrossRef ] [ Google Scholar ]
- Monti J. M., Monti D. (2007). The Involvement of Dopamine in the Modulation of Sleep and Waking . Sleep Med. Rev. 11 ( 2 ), 113–133. 10.1016/j.smrv.2006.08.003 [ PubMed ] [ CrossRef ] [ Google Scholar ]
- Munch M., Knoblauch V., Blatter K., Schroder C., Schnitzler C., Krauchi K., et al. (2004). The Frontal Predominance in Human EEG delta Activity after Sleep Loss Decreases with Age . Eur. J. Neurosci. 20 ( 5 ), 1402–1410. 10.1111/j.1460-9568.2004.03580.x [ PubMed ] [ CrossRef ] [ Google Scholar ]
- Munch M., Knoblauch V., Blatter K., Wirz-Justice A., Cajochen C. (2007). Is Homeostatic Sleep Regulation under Low Sleep Pressure Modified by Age . Sleep 30 ( 6 ), 781–792. 10.1093/sleep/30.6.781 [ PMC free article ] [ PubMed ] [ CrossRef ] [ Google Scholar ]
- Murray G., Allen N. B., Trinder J. (2002). Mood and the Circadian System: Investigation of a Circadian Component in Positive Affect . Chronobiology Int. 19 ( 6 ), 1151–1169. 10.1081/cbi-120015956 [ PubMed ] [ CrossRef ] [ Google Scholar ]
- Murray G., Nicholas C. L., Kleiman J., Dwyer R., Carrington M. J., Allen N. B., et al. (2009). Nature's Clocks and Human Mood: the Circadian System Modulates Reward Motivation . Emotion 9 ( 5 ), 705–716. 10.1037/a0017080 [ PubMed ] [ CrossRef ] [ Google Scholar ]
- Muto V., Jaspar M., Meyer C., Kussé C., Chellappa S. L., Degueldre C., et al. (2016). Local Modulation of Human Brain Responses by Circadian Rhythmicity and Sleep Debt . Science 353 ( 6300 ), 687–690. 10.1126/science.aad2993 [ PubMed ] [ CrossRef ] [ Google Scholar ]
- Nejad A. B., Fossati P., Lemogne C. (2013). Self-referential Processing, Rumination, and Cortical Midline Structures in Major Depression . Front. Hum. Neurosci. 7 , 666. 10.3389/fnhum.2013.00666 [ PMC free article ] [ PubMed ] [ CrossRef ] [ Google Scholar ]
- Nelson C. S., Dell’Angela K., Jellish S. W., Brown I. E., Skaredoff M. (1995). Residents' Performance before and after Night Call as Evaluated by an Indicator of Creative Thought . J. Am. Osteopath Assoc. 95 ( 10 ), 600. 10.7556/jaoa.1995.95.10.600 [ PubMed ] [ CrossRef ] [ Google Scholar ]
- Niendam T. A., Laird A. R., Ray K. L., Dean Y. M., Glahn D. C., Carter C. S. (2012). Meta-analytic Evidence for a Superordinate Cognitive Control Network Subserving Diverse Executive Functions . Cogn. Affect Behav. Neurosci. 12 ( 2 ), 241–268. 10.3758/s13415-011-0083-5 [ PMC free article ] [ PubMed ] [ CrossRef ] [ Google Scholar ]
- Niggemyer K. A., Begley A., Monk T., Buysse D. J. (2004). Circadian and Homeostatic Modulation of Sleep in Older Adults during a 90-minute Day Study . Sleep 27 ( 8 ), 1535–1541. 10.1093/sleep/27.8.1535 [ PubMed ] [ CrossRef ] [ Google Scholar ]
- Nilsson J. P., Soderstrom M., Karlsson A. U., Lekander M., Akerstedt T., Lindroth N. E., et al. (2005). Less Effective Executive Functioning after One Night's Sleep Deprivation . J. Sleep Res. 14 ( 1 ), 1–6. 10.1111/j.1365-2869.2005.00442.x [ PubMed ] [ CrossRef ] [ Google Scholar ]
- Pace-Schott E. F., Nave G., Morgan A., Spencer R. M. C. (2012). Sleep-dependent Modulation of Affectively Guided Decision-Making . J. Sleep Res. 21 ( 1 ), 30–39. 10.1111/j.1365-2869.2011.00921.x [ PMC free article ] [ PubMed ] [ CrossRef ] [ Google Scholar ]
- Perlis M. L., Grandner M. A., Brown G. K., Basner M., Chakravorty S., Morales K. H., et al. (2016a). Nocturnal Wakefulness as a Previously Unrecognized Risk Factor for Suicide . J. Clin. Psychiatry 77 ( 6 ), e726–e733. 10.4088/JCP.15m10131 [ PMC free article ] [ PubMed ] [ CrossRef ] [ Google Scholar ]
- Perlis M. L., Grandner M. A., Chakravorty S., Bernert R. A., Brown G. K., Thase M. E. (2016b). Suicide and Sleep: Is it a Bad Thing to Be Awake when Reason Sleeps . Sleep Med. Rev. 29 , 101–107. 10.1016/j.smrv.2015.10.003 [ PMC free article ] [ PubMed ] [ CrossRef ] [ Google Scholar ]
- Pigeon W. R., Pinquart M., Conner K. (2012). Meta-analysis of Sleep Disturbance and Suicidal Thoughts and Behaviors . J. Clin. Psychiatry 73 ( 9 ), e1160–e1167. 10.4088/JCP.11r07586 [ PubMed ] [ CrossRef ] [ Google Scholar ]
- Preti A., Miotto P. (2001). Diurnal Variations in Suicide by Age and Gender in Italy . J. Affective Disord. 65 ( 3 ), 253–261. 10.1016/s0165-0327(00)00232-9 10.1016/s0165-0327(00)00232-9 [ PubMed ] [ CrossRef ] [ CrossRef ] [ Google Scholar ]
- Reeve S., Emsley R., Sheaves B., Freeman D. (2018). Disrupting Sleep: The Effects of Sleep Loss on Psychotic Experiences Tested in an Experimental Study with Mediation Analysis . Schizophr Bull. 44 ( 3 ), 662–671. 10.1093/schbul/sbx103 [ PMC free article ] [ PubMed ] [ CrossRef ] [ Google Scholar ]
- Reeve S., Sheaves B., Freeman D. (2015). The Role of Sleep Dysfunction in the Occurrence of Delusions and Hallucinations: A Systematic Review . Clin. Psychol. Rev. 42 , 96–115. 10.1016/j.cpr.2015.09.001 [ PMC free article ] [ PubMed ] [ CrossRef ] [ Google Scholar ]
- Satterfield B. C., Raikes A. C., Killgore W. D. S. (2018). Rested-Baseline Responsivity of the Ventral Striatum Is Associated with Caloric and Macronutrient Intake during One Night of Sleep Deprivation . Front. Psychiatry 9 , 749. 10.3389/fpsyt.2018.00749 [ PMC free article ] [ PubMed ] [ CrossRef ] [ Google Scholar ]
- Schuck A., Calati R., Barzilay S., Bloch‐Elkouby S., Galynker I. (2019). Suicide Crisis Syndrome: A Review of Supporting Evidence for a New Suicide‐specific Diagnosis . Behav. Sci. L. 37 ( 3 ), 223–239. 10.1002/bsl.2397 [ PubMed ] [ CrossRef ] [ Google Scholar ]
- Sheaves B., Bebbington P. E., Goodwin G. M., Harrison P. J., Espie C. A., Foster R. G., et al. (2016). Insomnia and Hallucinations in the General Population: Findings from the 2000 and 2007 British Psychiatric Morbidity Surveys . Psychiatry Res. 241 , 141–146. 10.1016/j.psychres.2016.03.055 [ PMC free article ] [ PubMed ] [ CrossRef ] [ Google Scholar ]
- Shechter A., Grandner M. A., St-Onge M.-P. (2014). The Role of Sleep in the Control of Food Intake . Am. J. Lifestyle Med. 8 ( 6 ), 371–374. 10.1177/1559827614545315 [ PMC free article ] [ PubMed ] [ CrossRef ] [ Google Scholar ]
- Sheline Y. I., Barch D. M., Price J. L., Rundle M. M., Vaishnavi S. N., Snyder A. Z., et al. (2009). The Default Mode Network and Self-Referential Processes in Depression . Pnas 106 ( 6 ), 1942–1947. 10.1073/pnas.0812686106 [ PMC free article ] [ PubMed ] [ CrossRef ] [ Google Scholar ]
- Shenhav A., Cohen J. D., Botvinick M. M. (2016). Dorsal Anterior Cingulate Cortex and the Value of Control . Nat. Neurosci. 19 ( 10 ), 1286–1291. 10.1038/nn.4384 [ PubMed ] [ CrossRef ] [ Google Scholar ]
- Sisti D., Rocchi M. B. L., Macciò A., Preti A. (2012). The Epidemiology of Homicide in Italy by Season, Day of the Week and Time of Day . Med. Sci. L. 52 ( 2 ), 100–106. 10.1258/msl.2011.010147 [ PubMed ] [ CrossRef ] [ Google Scholar ]
- Slama H., Chylinski D. O., Deliens G., Leproult R., Schmitz R., Peigneux P. (2018). Sleep Deprivation Triggers Cognitive Control Impairments in Task-Goal Switching . Sleep 41 ( 2 ), zsx200. 10.1093/sleep/zsx200 [ PubMed ] [ CrossRef ] [ Google Scholar ]
- Stunkard A. J., Allison K. C., Lundgren J. D., O'Reardon J. P. (2009). A Biobehavioural Model of the Night Eating Syndrome . Obes. Rev. 10 ( Suppl. 2 ), 69–77. 10.1111/j.1467-789X.2009.00668.x [ PubMed ] [ CrossRef ] [ Google Scholar ]
- Ten Thij M., Bathina K., Rutter L. A., Lorenzo-Luaces L., van de Leemput I. A., Scheffer M., et al. (2020). Depression Alters the Circadian Pattern of Online Activity . Sci. Rep. 10 ( 1 ), 17272. 10.1038/s41598-020-74314-3 [ PMC free article ] [ PubMed ] [ CrossRef ] [ Google Scholar ]
- Tononi G., Cirelli C. (2019). Sleep and Synaptic Down‐selection . Eur. J. Neurosci. 51 , 413–421. 10.1111/ejn.14335 [ PMC free article ] [ PubMed ] [ CrossRef ] [ Google Scholar ]
- Toyoda H., Li X.-Y., Wu L.-J., Zhao M.-G., Descalzi G., Chen T., et al. (2011). Interplay of Amygdala and Cingulate Plasticity in Emotional Fear . Neural Plasticity 2011 , 1–9. 10.1155/2011/813749 [ PMC free article ] [ PubMed ] [ CrossRef ] [ Google Scholar ]
- Tubbs A. S., Fernandez F.-X., Johnson D. A., Perlis M. L., Grandner M. A. (2021). Nocturnal and Morning Wakefulness Are Differentially Associated with Suicidal Ideation in a Nationally Representative Sample . J. Clin. Psychiatry 82 ( 6 ), 20m13820. 10.4088/JCP.20m13820 [ PubMed ] [ CrossRef ] [ Google Scholar ]
- Tubbs A. S., Fernandez F.-X., Perlis M. L., Hale L., Branas C. C., Barrett M., et al. (2020a). Suicidal Ideation Is Associated with Nighttime Wakefulness in a Community Sample . Sleep 44 , zsaa128. 10.1093/sleep/zsaa128 [ PMC free article ] [ PubMed ] [ CrossRef ] [ Google Scholar ]
- Tubbs A. S., Perlis M. L., Basner M., Chakravorty S., Khader W., Fernandez F., et al. (2020b). Relationship of Nocturnal Wakefulness to Suicide Risk across Months and Methods of Suicide . J. Clin. Psychiatry 81 ( 2 ), 19m12964. 10.4088/JCP.19m12964 [ PMC free article ] [ PubMed ] [ CrossRef ] [ Google Scholar ]
- van Houwelingen C. A., Beersma D. G. (2001). Seasonal Changes in 24-h Patterns of Suicide Rates: a Study on Train Suicides in The Netherlands . J. Affect Disord. 66 ( 2-3 ), 215–223. 10.1016/s0165-0327(00)00308-6 10.1016/s0165-0327(00)00308-6 [ PubMed ] [ CrossRef ] [ CrossRef ] [ Google Scholar ]
- Vander Wal J. S. (2012). Night Eating Syndrome: a Critical Review of the Literature . Clin. Psychol. Rev. 32 ( 1 ), 49–59. 10.1016/j.cpr.2011.11.001 [ PubMed ] [ CrossRef ] [ Google Scholar ]
- Venkatraman V., Chuah Y. L., Huettel S. A., Chee M. W. (2007). Sleep Deprivation Elevates Expectation of Gains and Attenuates Response to Losses Following Risky Decisions . Sleep 30 ( 5 ), 603–609. 10.1093/sleep/30.5.603 [ PubMed ] [ CrossRef ] [ Google Scholar ]
- Venkatraman V., Huettel S. A., Chuah L. Y. M., Payne J. W., Chee M. W. L. (2011). Sleep Deprivation Biases the Neural Mechanisms Underlying Economic Preferences . J. Neurosci. 31 ( 10 ), 3712–3718. 10.1523/JNEUROSCI.4407-10.2011 [ PMC free article ] [ PubMed ] [ CrossRef ] [ Google Scholar ]
- Verweij I. M., Romeijn N., Smit D. J., Piantoni G., Van Someren E. J., van der Werf Y. D. (2014). Sleep Deprivation Leads to a Loss of Functional Connectivity in Frontal Brain Regions . BMC Neurosci. 15 , 88. 10.1186/1471-2202-15-88 [ PMC free article ] [ PubMed ] [ CrossRef ] [ Google Scholar ]
- Volkow N. D., Tomasi D., Wang G.-J., Telang F., Fowler J. S., Logan J., et al. (2012). Evidence that Sleep Deprivation Downregulates Dopamine D2R in Ventral Striatum in the Human Brain . J. Neurosci. 32 ( 19 ), 6711–6717. 10.1523/JNEUROSCI.0045-12.2012 [ PMC free article ] [ PubMed ] [ CrossRef ] [ Google Scholar ]
- Volkow N. D., Wang G.-J., Telang F., Fowler J. S., Logan J., Wong C., et al. (2008). Sleep Deprivation Decreases Binding of [11C]raclopride to Dopamine D2/D3 Receptors in the Human Brain . J. Neurosci. 28 ( 34 ), 8454–8461. 10.1523/JNEUROSCI.1443-08.2008 [ PMC free article ] [ PubMed ] [ CrossRef ] [ Google Scholar ]
- Vollen K. H., Watson C. G. (1975). Suicide in Relation to Time of Day and Day of Week . AJN, Am. J. Nurs. 75 ( 2 ), 263. 10.1097/00000446-197502000-00018 [ PubMed ] [ CrossRef ] [ Google Scholar ]
- Voros V., Tenyi T., Nagy A., Fekete S., Osvath P. (2021). Crisis Concept Re-loaded?-the Recently Described Suicide-specific Syndromes May Help to Better Understand Suicidal Behavior and Assess Imminent Suicide Risk More Effectively . Front. Psychiatry 12 , 598923. 10.3389/fpsyt.2021.598923 [ PMC free article ] [ PubMed ] [ CrossRef ] [ Google Scholar ]
- Williams P., Tansella M. (1987). The Time for Suicide . Acta Psychiatr. Scand. 75 ( 5 ), 532–535. 10.1111/j.1600-0447.1987.tb02829.x [ PubMed ] [ CrossRef ] [ Google Scholar ]
- Wimmer F., Hoffmann R. F., Bonato R. A., Moffitt A. R. (1992). The Effects of Sleep Deprivation on Divergent Thinking and Attention Processes . J. Sleep Res. 1 ( 4 ), 223–230. 10.1111/j.1365-2869.1992.tb00043.x [ PubMed ] [ CrossRef ] [ Google Scholar ]
- Wirz-Justice A. (2008). Diurnal Variation of Depressive Symptoms . Dialogues Clin. Neurosci. 10 ( 3 ), 337–343. [ PMC free article ] [ PubMed ] [ Google Scholar ]
- Womack S. D., Hook J. N., Reyna S. H., Ramos M. (2013). Sleep Loss and Risk-Taking Behavior: a Review of the Literature . Behav. Sleep Med. 11 ( 5 ), 343–359. 10.1080/15402002.2012.703628 [ PubMed ] [ CrossRef ] [ Google Scholar ]
- Woo E., Sansing L. H., Arnsten A. F. T., Datta D. (2021). Chronic Stress Weakens Connectivity in the Prefrontal Cortex: Architectural and Molecular Changes . Chronic Stress 5 , 247054702110292. (Thousand Oaks). 10.1177/24705470211029254 [ PMC free article ] [ PubMed ] [ CrossRef ] [ Google Scholar ]
- Wright K. P., Lowry C. A., Lebourgeois M. K. (2012). Circadian and Wakefulness-Sleep Modulation of Cognition in Humans . Front. Mol. Neurosci. 5 , 50. 10.3389/fnmol.2012.00050 [ PMC free article ] [ PubMed ] [ CrossRef ] [ Google Scholar ]
- Yoshimura S., Okamoto Y., Onoda K., Matsunaga M., Ueda K., Suzuki S.-i., et al. (2010). Rostral Anterior Cingulate Cortex Activity Mediates the Relationship between the Depressive Symptoms and the Medial Prefrontal Cortex Activity . J. Affective Disord. 122 ( 1-2 ), 76–85. 10.1016/j.jad.2009.06.017 [ PubMed ] [ CrossRef ] [ Google Scholar ]
- Zhang Y., Ren R., Sanford L. D., Yang L., Zhou J., Zhang J., et al. (2019). Sleep in Posttraumatic Stress Disorder: A Systematic Review and Meta-Analysis of Polysomnographic Findings . Sleep Med. Rev. 48 , 101210. 10.1016/j.smrv.2019.08.004 [ PubMed ] [ CrossRef ] [ Google Scholar ]
'IF': How John Krasinski's daughters helped him create his 'most personal' movie yet

When John Krasinski was 8 years old, he wanted braces more than anything.
That desire was short-lived. ("Turns out, braces aren't as comfortable or as fun as you thought they'd be," Krasinski quips.) But it did spark the name of his imaginary friend, Sam Brace, who would trek with him to the video store near his boyhood home in the Boston suburbs. There, Krasinski picked out the latest action films and comedies, which he proceeded to act out with Sam.
“We would live through these movies,” recalls " The Office " star, who wrote and directed the new family adventure “IF” (in theaters Friday), an acronym for “imaginary friend.” During a recent interview, “someone said, ‘So, Sam directed this movie.’ And I totally blacked out and went, ‘Oh, my God. Sam totally did.’”
John Krasinski and wife Emily Blunt wanted to make 'IF' for their daughters
Not everyone has such fond memories of their imaginary friends. Steve Carell , who plays a giant purple IF named Blue, only vaguely remembers having one.
Need a break? Play the USA TODAY Daily Crossword Puzzle.
"I’m hoping to reclaim that friendship," Carell deadpans. "Perhaps this movie will send me in that direction.”
Cailey Fleming, who portrays the film’s young protagonist Bea, similarly never had pretend pals growing up. But she says she's "never been so moved by a script before."
“I couldn't get through the whole thing without bawling,” says the 17-year-old actress (" The Walking Dead "). “And that's something John and I have in common: We both cry very easily.”
“IF” begins with a heart-wrenching montage in which a younger Bea (Audrey Hoffman) loses her ailing mom (Catharine Daddario). Years later and now a preteen, Bea is sent to stay with her grandmother (Fiona Shaw) while her dad (Krasinski) undergoes surgery. Lonely and wandering around her grandma’s apartment building, she meets a mysterious man (Ryan Reynolds) and a throng of magical creatures, which turn out to be retired IFs whose kids forgot about them when they grew up.
Krasinski, 44, was inspired to make the movie during the pandemic, while sheltering with his wife, Oscar nominee Emily Blunt , and their two daughters, Hazel (10) and Violet (7).
“All the imaginary games that my girls were playing became fewer and fewer,” Krasinski says. “I genuinely saw their lights starting to go out, and they started asking big questions like, ‘Are we going to be OK?’ I said to Emily, ‘This is the definition of growing up,' when you start to make that choice of, ‘Do I let go of all this childhood stuff to be in your real world?’ ”
In writing this story, he wanted to show that "you don't have to choose. That magical world you created is a time capsule you can always go back to."
'IF' is an unexpected 'companion' piece to 'A Quiet Place'
For a visual-effects-heavy kids' movie, "IF" feels remarkably low stakes, as Bea helps find new homes for the imaginary friends and impels adults to reconnect with their inner child. Thankfully, Krasinski says, there was no pressure for a "big action sequence." Rather, he took cues from the films that moved him most as a child, including “E.T.,” “The Goonies” and “Dead Poets Society."
Growing up, "those filmmakers actually gave me credit that I could hold bigger themes," Krasinski says. "That’s really what I took with me into this movie: Don’t shy away from emotionality.”
Although they couldn’t be more different, “IF” has unlikely parallels to his 2018 sci-fi thriller “A Quiet Place,” about a family fighting for survival after an alien invasion. For "Quiet Place," Krasinski drew from his own anxieties as a dad wanting to protect his kids. But "IF" turns the tables to focus on children's worries and fears.
“I love the idea of them being companion pieces,” Krasinski says. "They're both about love and family." Blunt had just given birth to Violet before the first "Quiet Place" film, so “we were in that new parent zone and that felt very authentic. This feels even more authentic," in that "I got this experience of working with my kids and talking to my kids and having them be a part of this from the beginning. It was the most personal thing I have ever done in my career."
Steve Carell says it was 'a no-brainer' reuniting with his 'The Office' co-star
Throughout the making of “IF,” Krasinski’s daughters weighed in on every aspect of the film: He showed them early sketches he drew of the various IFs, and they offered feedback on which scenes to use in the trailer. Their imaginary friends even became characters in the movie, voiced by Maya Rudolph and Krasinski, respectively.
“The pink alligator is Violet’s imaginary friend, and Hazel’s imaginary friend is the marshmallow that lights on fire,” Krasinski explains. “Hazel is a very empathetic person. We were making s'mores one day and her marshmallow caught on fire, and she was emotionally destroyed.”
The movie features an A-list roster of celebrities playing the IFs, including Bradley Cooper , Phoebe Waller-Bridge , George Clooney , Matt Damon and Blake Lively . To portray Blue, Krasinski knew he needed someone who was "sweet" but also "vulnerable." He immediately turned to his beloved "Office" co-star Carell, 61, who has also been his longtime go-to for parenting advice, as a dad to Annie (22) and John (19) with wife Nancy Walls.
"We love each other and we've always worked well together," Carell says. "John is a fantastic director, and there's a reason everybody jumped at the chance to be in this movie. It was a no-brainer for me."
The actors light up when talking about kids' vivid imaginations. Carell is mildly traumatized by this reporter's childhood belief that lobsters lived in the toilet, just waiting to pull us in.
"Now I will always feel like there's a lobster in my toilet," Carell says with a laugh. Growing up, "my son thought that when I was young, the world was in black and white. He didn't think color appeared in the world until he was on the scene. So that's how old he thinks I am!"
Arizona's best places for night owls: These 3 cities made the after-dark list

As temperatures heat up in Arizona, evening activities might increase too. For those seeking things to do after dark when it's a little cooler, there's good news: Three Arizona nightlife destinations made a list of the top 150 in the nation.
A recent ranking by photobook company Mixbook analyzed the best places for night owls in the United States. Whether you like wandering through bustling night markets, grabbing a midnight premiere at a local cinema or lingering in 24-hour diners that serve breakfast at midnight, many cities cater to the nocturnal culture, the study found.
In Arizona, Roosevelt Row, Old Town Scottsdale and Tucson's Historic Fourth Avenue made the cut.
Here's why these Arizona spots cracked the list, as well as the top 10 places for night owls in the U.S.
Top Arizona nightlife spots
Mixbook surveyed 3,000 seasoned travelers to find the top areas in the U.S. for nocturnal life. These three in Arizona were named among the country's best.
Need a break? Play the USA TODAY Daily Crossword Puzzle.
- Roosevelt Row: This downtown Phoenix nightlife hotspot features a mix of stylish bars and eateries as well as the First Fridays art walk and other cultural events. Roosevelt Row's dynamic atmosphere makes it a prime destination for night activities, placing it 32nd in the Mixbook ranking.
- Old Town Scottsdale: With an array of live music venues and trendy lounges and restaurants , Old Town Scottsdale has everything from craft beer pubs to Western saloons. It ranked 41 on the list.
- Downtown Tucson/Historic Fourth Avenue: Known for its bohemian flair, historic charm and vibrant community, Fourth Avenue ranked 117 on the list. This neighborhood is home to eclectic shops and restaurants as well as the Historic Fourth Avenue Street Fair, which draws visitors from all over the state.
Top 10 nightlife spots in the U.S.
These are the nation's 10 best places for night owls, according to Mixbook:
- Downtown Las Vegas.
- French Quarter, New Orleans.
- Kailua-Kona, Big Island, Hawaii.
- SoHo, Manhattan.
- Downtown Anchorage, Alaska.
- Savannah Historic District, Georgia.
- Old Colorado City, Colorado Springs.
- Downtown Nashville.
- Deep Ellum, Dallas.
- Waikiki, Honolulu.
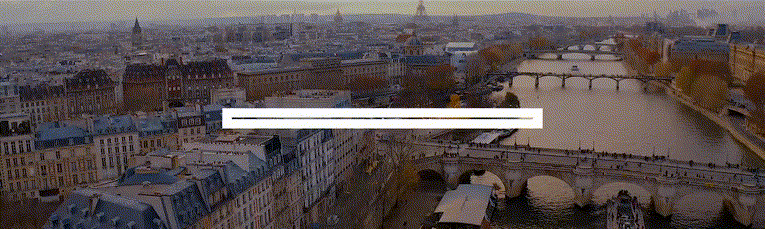
IMAGES
VIDEO
COMMENTS
The term "sundowning" refers to a state of confusion that occurs in the late afternoon and lasts into the night. Sundowning can cause various behaviors, such as confusion, anxiety, aggression or ignoring directions. Sundowning also can lead to pacing or wandering. Sundowning isn't a disease. It's a group of symptoms that occurs at a specific ...
To improve the definition of the disorder and establish diagnostic criteria with levels of certainty, a consensus conference using formal recommended methodology was held in Bologna in September 2014. ... protracted ambulatory behavior known as epileptic nocturnal wandering. 17,22,24,25 These events can last longer than 2 minutes.
Wandering behavior is one of the most important and challenging management aspects in persons with dementia. Wandering behavior in people with dementia (PwD) is associated with an increased risk of falls, injuries, and fractures, as well as going missing or being lost from a facility. This causes increased distress in caregivers at home and in ...
As noted by the Ohayon et al. [1], nocturnal wandering (NW) is not synonymous with sleepwalking. NW may also refer to wandering during the night due to epilepsy. Alcohol intoxication is well known to result in drunken behavior while awake but this type of cognitive impairment may be undistinguishable from other forms of NW. Dementia and CNS ...
The definition of wandering varies from context to context. Technically, wandering is an umbrella term for several different behaviors. This includes elopement (attempts to escape), repetitive ...
Also called "sundowner's syndrome," it is not a disease but a set of symptoms or dementia-related behaviors that may include difficulty sleeping, anxiety, agitation, hallucinations, pacing and disorientation. Although the exact cause is unknown, sundowning may occur due to disease progression and changes in the brain.
Consequently, it seems unlikely that these medications cause nocturnal wandering, but rather that they appear to trigger events in predisposed individuals. We did find a higher risk of frequent nocturnal wandering episodes among individuals with insomnia disorder but not with hypnotic intake (benzodiazepine or benzodiazepine-like hypnotics).
Madson J (2016) The study of wandering in persons with senile dementia, American Journal of Alzheimer's Care and Related Disorders & Research, 10.1177/153331759100600105, 6:1, (21-24), Online publication date: 1-Jan-1991.
Episodic Nocturnal Wandering (ENW): Preceded by both PA and NPD, episodic nocturnal wandering results in agitated wandering outside the bed. These episodes are the rarest nocturnal seizure subtype, only occurring in ~2% of nocturnal seizures. There may be some symptoms resembling dysautonomia with screaming, yelling, or talking and there still ...
Wandering. Alzheimer's disease causes people to lose their ability to recognize familiar places and faces. It's common for a person living with dementia to wander or become lost or confused about their location, and it can happen at any stage of the disease. Six in 10 people living with dementia will wander at least once; many do so repeatedly.
Objective: To assess the prevalence and comorbid conditions of nocturnal wandering with abnormal state of consciousness (NW) in the American general population. Methods: Cross-sectional study conducted with a representative sample of 19,136 noninstitutionalized individuals of the U.S. general population ≥18 years old. The Sleep-EVAL expert system administered questions on life and sleeping ...
Ohayon M, Mahowald M, Dauvilliers Y, Krystal A, Leger D. Prevalence and comorbidity of nocturnal wandering in the US adult general population. Neurology 2012;78:1583-9. Schenck C, Milner D, Hurwitz T, Bundlie S, Mahowald M. A polysomnographic and clinical report on sleep related injury in 100 adult patients. Am J Psychiatry 1989;146:1166-73.
The lifetime prevalence of nocturnal wandering with abnormal state of consciousness among US adults may be as high as 29.2%. [ 17 ] ; however, the prevalence of sleepwalking disorder, marked by repeated episodes and impairment or distress, is much lower (1-5%).
To improve the definition of the disorder and establish diagnostic criteria with levels of certainty, a consensus conference using formal recommended methodology was held in Bologna in September 2014. ... protracted ambulatory behavior known as epileptic nocturnal wandering. 17, 22, 24, 25 These events can last longer than 2 minutes.
A randomly selected group of patients with a clinical history of paroxysmal arousal, nocturnal wandering or hyperkinetic seizures, composed the SHE group , ... Hesdorffer D, Kahane P, Nobili L, et al. Definition and diagnostic criteria of sleep-related hypermotor epilepsy. Neurology. (2016) 86:1834-42. doi: 10.1212/WNL.0000000000002666.
Occasionally patients may jump out of bed and run around in a repetitive manner, a phenomenon was named Epileptic Nocturnal Wandering (ENW) [18] and difficult to distinguish from sleep-walking even for experts in sleep medicine and epileptology. The second feature of HS is an asymmetric tonic-dystonic posturing usually with strong autonomic ...
Abstract. As noted by Ohayon et al., 1 nocturnal wandering (NW) is not synonymous with sleepwalking. NW may also refer to wandering during the night due to epilepsy. Alcohol intoxication can also result in drunken behavior while awake, but this type of cognitive impairment may be undistinguishable from other forms of NW.
The sleep disturbance that is characterized by nocturnal wandering and confusion: Night time: Not reported: Not reported: Yes: Primary sleep disorder: Yes: Not reported: 12%: Not reported: Diagnostic manual: Volicer et al., 2001, [18] ... There is no consensus in the definition in the scientific community; literature is divided on the existence ...
In the following years, a large series of patients were described.10,11 A large video-polysomnographic study of 100 consecutive NFLE cases highlighted the different intensity and durations of these sleep-related manifestations (ranging from paroxysmal arousals to nocturnal wandering) that could occur in a single patient, during a single night.
Seizures happen when there is unusual electrical activity in the brain. Seizures that occur during sleep are called nocturnal seizures. Nocturnal seizures can disrupt sleep and cause poor sleep quality and excessive sleepiness. Occasionally, people can be injured or even die during nocturnal seizures. Learning more about nocturnal seizures ...
Sleep-related hypermotor epilepsy (SHE), formerly known as Nocturnal Frontal Lobe Epilepsy is a focal epilepsy characterized by seizures with complex hyperkinetic automatisms and/or asymmetric tonic/dystonic posturing occurring mostly during sleep. SHE is a rare disease with an estimated minimum prevalence of 1.8/100,000 individuals and represent about 10% of drug-resistant surgical cases ...
WANDERING definition: 1. present participle of wander 2. to walk around slowly in a relaxed way or without any clear…. Learn more.
"Whether it's lingering in 24-hour diners that serve breakfast at midnight, wandering through bustling night markets, or catching a midnight premiere at a local cinema, America's cities cater to ...
Sufficient sleep with minimal interruption during the circadian/biological night supports daytime cognition and emotional regulation. Conversely, disrupted sleep involving significant nocturnal wakefulness leads to cognitive and behavioral dysregulation. Most studies to-date have examined how fragmented or insufficient sleep affects next-day ...
"The Office" star John Krasinski reunites with Steve Carell on his "most personal" movie yet: "IF," a kid-friendly follow-up to "A Quiet Place."
These are the nation's 10 best places for night owls, according to Mixbook: Downtown Las Vegas. French Quarter, New Orleans. Kailua-Kona, Big Island, Hawaii. SoHo, Manhattan.