- Sustainable
- Energy Economy
- Energy Services

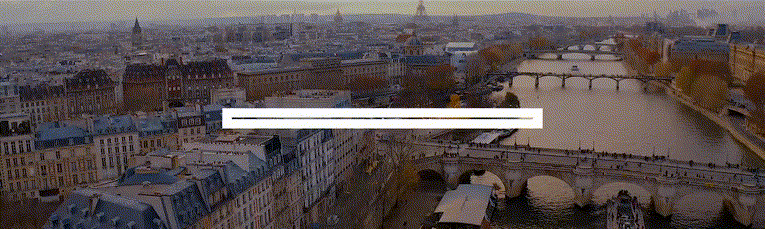
What is Round Trip Efficiency?
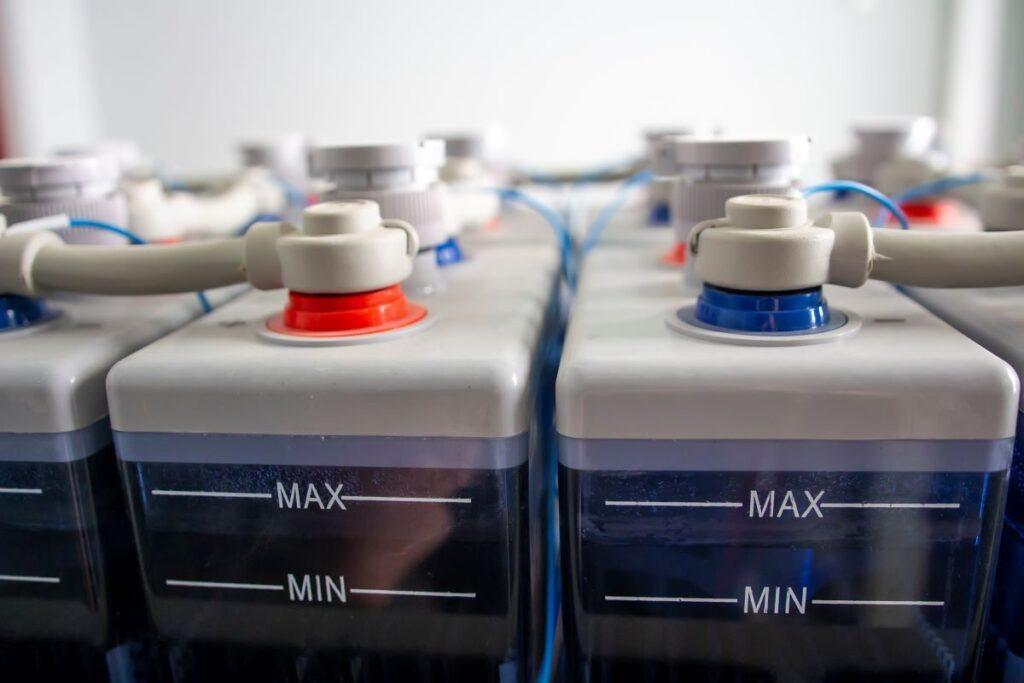
Energy storage systems function by taking in electricity, storing it, and subsequently returning it to the grid. The round trip efficiency (RTE), also known as AC/AC efficiency, refers to the ratio between the energy supplied to the storage system (measured in MWh) and the energy retrieved from it (also measured in MWh). This efficiency is expressed as a percentage (%).
The round trip efficiency is a crucial factor in determining the effectiveness of storage technology. A higher RTE indicates that there is less energy loss during the storage process, resulting in a more efficient overall system. Grid systems engineers strive for energy storage systems to achieve an 80% RTE whenever feasible, as it signifies a desirable level of efficiency and minimizes energy losses.
What Factors Can Affect the Round Trip Efficiency of an Energy Storage System?
The RTE of an energy storage system can be influenced by various factors, including:
1. Technology: Different storage technologies have varying round-trip efficiencies. For example, hydro storage typically ranges from 65% in older installations to 75-80% in modern deployments, while flywheels have efficiencies of about 80% to 90%. Some battery technologies can have round-trip efficiencies ranging from 75% to 90%.
2. Storage duration: Some technologies may experience leakage or energy loss over long-term storage, which can affect round-trip efficiency. It is important to consider the specific characteristics and limitations of the storage technology when evaluating its efficiency.
3. Age and condition of the system: Older storage systems may have lower round-trip efficiencies compared to newer ones. Factors such as wear and tear, component degradation, and maintenance practices can impact the overall efficiency of the system.
4. Charging and discharging rates: The speed at which energy is charged into and discharged from the storage system can affect its efficiency. Certain technologies may have lower efficiencies at high charging or discharging rates.
5. System design and control: The design and control strategies implemented in the energy storage system can influence its round-trip efficiency. Optimal system design, efficient power electronics, and effective control algorithms can improve the overall efficiency of the system.
6. Temperature: Temperature can have an impact on the performance and efficiency of energy storage systems. Extreme temperatures can affect the efficiency of certain storage technologies, such as batteries, leading to lower round-trip efficiencies.
Considering these factors is crucial when evaluating the round-trip efficiency of an energy storage system, as they can significantly affect its performance and effectiveness in storing and retrieving energy.
Must Read: What is Power Conversion Efficiency?
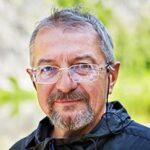
Elliot is a passionate environmentalist and blogger who has dedicated his life to spreading awareness about conservation, green energy, and renewable energy. With a background in environmental science, he has a deep understanding of the issues facing our planet and is committed to educating others on how they can make a difference.
Related Posts
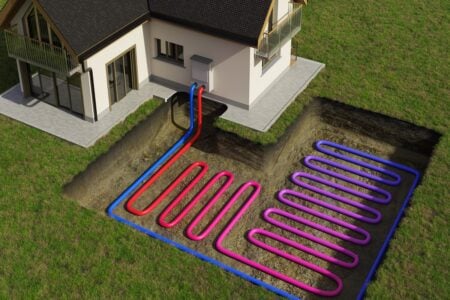
What is Heating Seasonal Performance Factor (HSPF)?
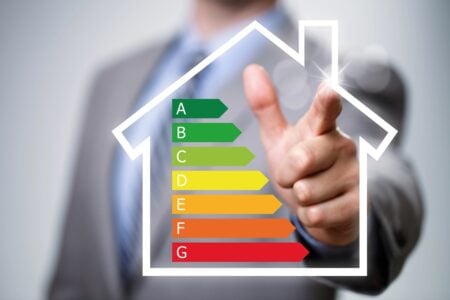
What is Annual Fuel Utilization Efficiency (AFUE)?

What is Grid Parity?
Save my name, email, and website in this browser for the next time I comment.
Type above and press Enter to search. Press Esc to cancel.
- POWER Plant ID
- POWER Events
- Connected Plant
- Distributed Energy
- International
- COVID-19 Coverage
- Carbon Capture
- Climate change
- Cybersecurity
- Distributed Power
- Electric Vehicles
- Energy Storage
- Environmental
- Instrumentation & Controls
- Legal & Regulatory
- Legislative
- Ocean/Marine
- Physical security
- Plant Design
- Power Demand
- Research and Development
- Supply Chains
- Tidal Power
- Waste to Energy
- About POWER
- Privacy Policy
- Cookie Settings
- Diversity, Equity, Inclusion & Belonging
- Accessibility Statement
Don’t Neglect Round-Trip Efficiency and Cost of Charging When Considering Levelized Cost of Storage
The world is moving toward renewable sources for electricity generation in an attempt to reduce fossil-fuel reliance. But wind and solar can’t provide a consistent flow of power 24/7, and grid operators have realized that new electricity generation needs to be paired with storage to manage periods with no sun or wind.
The decreasing cost of lithium-ion batteries has made battery energy storage systems (BESS) more affordable; however, the cost of battery storage systems represents only 20%-25% of any project’s lifetime cost. Power equipment, land, site work, cabling, project design and management, grid integration, transportation, and other related up-front costs represent another 25%.
So, what makes up the other ~50%? Operations and maintenance, otherwise known as O&M, represent a few percentage points. O&M generally includes expenses associated with maintaining, repairing, and operating energy storage systems over their lifespan. The rest comes from the cost of electricity to charge the system, which is significantly affected by the system’s overall round-trip efficiency (RTE).
Why RTE and Cost of Energy Matter
Levelized cost of storage (LCOS) is a metric used to determine the cost per unit of energy discharged from an energy storage system. The calculation is usually expressed in dollars per megawatt hour (MWh) and includes initial costs plus operating costs divided by the energy discharged over the asset’s service life.
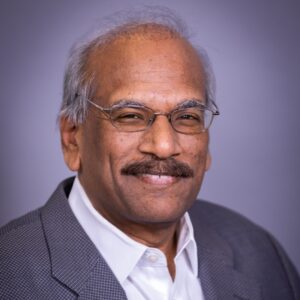
There are dozens of potential variables that may be used to determine the true levelized cost of storage, and different vendors will add, omit, or adjust different ones to put their products in the best light. This is why it’s so important to understand the role of RTE and cost of energy in a storage system, because they often have the biggest impact. These are also components that vendors with low-RTE technologies will most often discount (or omit altogether).
Round-trip efficiency is a measure of the amount of energy put into a system compared to the amount dispatched, and is expressed as a percentage. A system with a high RTE (75%+) is able to dispatch most of the energy fed into it. A low RTE indicates that the system loses a considerable amount of energy, often to heat arising from irreversible side reactions or high internal cell resistance. Many long-duration energy storage systems have RTEs below 50%, creating a significant amount of energy waste.
For example, lithium-ion batteries generally have RTEs of 90%+. In contrast, lead-acid batteries have lower RTEs of around 70%, meaning that approximately 30% of charge energy is lost. RTEs for flow batteries can range from 50%–75%, while metal-air batteries could have RTEs as low as 40%.
If the electricity used to charge low-RTE batteries was free, efficiency might not matter much. But electricity always comes with a cost. Some might argue that during periods where supply exceeds demand, renewables could be used to charge batteries when they would otherwise be curtailed. There’s a logic to that, but curtailment periods can’t always be predicted.
Even if you’re using electricity that would otherwise be curtailed, you have to assign a monetary value. If a turbine is spinning or a solar panel is generating electricity and a battery system is storing that electricity, every component in the system is subject to normal wear and tear plus maintenance and replacement protocols—all of which have costs associated. Factors at play include:
Technology lifespan and degradation rate. An energy storage system’s service life is determined by technology and cycles. All energy storage systems deteriorate over time, making them less efficient at storing and discharging energy. The same goes for generation sources. From solar to wind to flow batteries to lithium-ion, the more the components are used, the shorter the lifespan and the sooner the need for repair, replacement or augmentation.
Maintenance costs. Solar panels, wind turbines, battery systems, transmission lines, and power equipment all have to be maintained. The more they’re used, the more often components need to be serviced or replaced.
Long-Duration Doesn’t Always Mean Lower LCOS
The latest buzzy term in the energy space is “long-duration energy storage,” or LDES for short. While there’s no single definition of what the term means, the term has generally come to describe a non-lithium storage technology that can provide energy for anywhere from 8 to 160 hours at a lower installed cost per MW than lithium-ion batteries or a standard natural gas turbine.
LDES isn’t confined to battery storage; non-battery technologies include compressed air, latent heat, flywheels, and more. In fact, pumped hydro currently accounts for the vast majority of all LDES capacity in the US, and will likely remain in that position for an extended time. Battery technologies being positioned for LDES use include flow batteries, zinc-based chemistries, metal air, nickel hydrogen, and more.
These technologies all work well and are generally safer than lithium-ion batteries, but they come with trade-offs. Many have high up-front costs and must be amortized over 30–40-year periods to be cost competitive. Some have very low energy densities, requiring significant amounts of land for installations above a few megawatt hours. Some are rate-limited and can’t discharge as quickly as needed for specific applications. Some have very restricted siting requirements. And maybe most importantly, many have RTEs below 60%, with a few at 40% or lower.
So, what does this all mean? The race is on to build a better storage system, and with no universal standard for calculating LCOS, every vendor is using a model that plays to the strength of their own technology. If you’re investigating a new storage technology, be sure to ask a few questions when LCOS numbers come up, such as:
How many years are they calculating when it comes to system life? Lithium-ion batteries usually have to be augmented or replaced somewhere between 10 and 15 years of use; vendors with low densities or high installed costs may calculate over 30–40 years to lower their LCOS while factoring in two or more replacement cycles for lithium-ion.
What are they using for the cost of electricity to charge the system, and how does that compare with your actual costs? Even if you’re only planning to charge the system during periods you’d normally be curtailing renewables, remember that there’s still a cost to running those systems. A system with a low RTE may end up having a much higher LCOS even when you’re paying very little for electricity.
Are they including the cost of land in their calculations? If you’re installing a storage facility in a rural area where land is cheap, this may not matter so much. But if you need to place storage in or near a high-cost-of-living area, cost of land (and availability) could be one of your primary concerns and should definitely play a role in the LCOS calculation.
Are they including installation tax credits (ITCs) or production tax credits (PTCs) in their calculations? If so, be sure that the numbers are correct for your projects, and that the same are being applied to any other technologies you’re evaluating.
— Mukesh Chatter is the CEO of Alsym Energy , a technology company developing a low-cost, high-performance rechargeable battery chemistry that is free of lithium and cobalt.
SHARE this article

Today in Energy
- Recent articles
- liquid fuels
- natural gas
- electricity
- oil/petroleum
- production/supply
- consumption/demand
- exports/imports
- international
- forecasts/projections
- steo (short-term energy outlook)
Utility-scale batteries and pumped storage return about 80% of the electricity they store
Electric energy storage is becoming more important to the energy industry as the share of intermittent generating technologies, such as wind and solar, in the electricity mix increases. Electric energy storage helps to meet fluctuating demand, which is why it is often paired with intermittent sources. Storage technologies include batteries and pumped-storage hydropower , which capture energy and store it for later use. Storage metrics can help us understand the value of the technology. Round-trip efficiency is the percentage of electricity put into storage that is later retrieved. The higher the round-trip efficiency, the less energy is lost in the storage process. According to data from the U.S. Energy Information Administration (EIA), in 2019, the U.S. utility-scale battery fleet operated with an average monthly round-trip efficiency of 82%, and pumped-storage facilities operated with an average monthly round-trip efficiency of 79%.
EIA’s Power Plant Operations Report provides data on utility-scale energy storage, including the monthly electricity consumption and gross electric generation of energy storage assets, which can be used to calculate round-trip efficiency. The metrics reviewed here use the finalized data from the Power Plant Operations Report for 2019—the most recent year for which a full set of storage data is available.
Pumped-storage facilities are the largest energy storage resource in the United States. The facilities collectively account for 21.9 gigawatts (GW) of capacity and for 92% of the country’s total energy storage capacity as of November 2020.
In recent years, utility-scale battery capacity has grown rapidly as battery costs have decreased. As batteries have been increasingly paired with renewables , they have become the second-largest source of electricity storage. As of November 20, 2020, utility-scale battery capacity had 1.4 GW of operational capacity. Another 4.0 GW of battery capacity is scheduled to come online in 2021, according to EIA’s Preliminary Electric Generator Inventory .
Although battery storage has slightly higher round-trip efficiency than pumped storage, pumped-storage facilities typically operate at utilization factors that are currently twice as high as batteries. Increasing durations among battery applications could shift battery operations toward services that reward longer output periods. For example, in 2015, the weighted average battery duration was a little more than 46 minutes, but by 2019, weighted average battery durations had doubled to 1.5 hours. The role of batteries and their capability to provide high levels of round-trip efficiency may become more important as batteries continue to be deployed and as the intermittent renewables share of the electricity mix grows.
Tags: storage , electricity

- ORIENTATION
- Instructor Information
- RESS PROGRAM
- CREATING A PRESENTATION
- WRITING STYLE GUIDES
- Getting Help
10.2 Key Metrics and Definitions for Energy Storage

Key Metrics and Definitions for Energy Storage
There are a few key technical parameters that are used to characterize a specific storage technology or system. Those characteristics will determine compatibility of the storage with a proposed application and will also have impact on its economic feasibility. Let us go through some definitions.
Storage Capacity
Capacity essentially means how much energy maximum you can store in the system. For example, if a battery is fully charged, how many watt-hours are put in there? If the water reservoir in the pumped hydro storage system is filled to capacity, how many watt-hours can be generated by releasing that water? Those amounts are determined by storage capacity.
Understandably, the capacity of any storage will increase with the system size. The more battery stacks are installed, the more electric energy can be put in for storage. The larger the water reservoir, the greater energy turnaround becomes possible. The system size should be matched with the load and specific application.
Storage capacity is typically measured in units of energy: kilowatt-hours (kWh), megawatt-hours (MWh), or megajoules (MJ). You will typically see capacities specified for a particular facility with storage or as total installed capacities within an area or a country.
Sometimes you will see capacity of storage specified in units of power (watt and its multiples) and time (hours).
For example: 60 MW battery system with 4 hours of storage . What does it mean?
60 MW means that the system can generate electricity at the maximum power of 60 MW for 4 hours straight. That also means that the total amount of energy stored in the system is:
60 MW x 4 hours = 240 MWh
But it can also provide less power if needed. For example, if the load only requires 20 MW, the system can supply it for 12 hours. The total amount of stored energy is the same, but it is used more slowly:
20 MW x 12 hours = 240 MWh
So power and time ratings give us a little bit more information: we not only know how much energy is stored, but can also define at what maximum rate this energy can be potentially used.
Check Your Understanding Questions 1 & 2 (Multiple Choice)
Energy density.
Energy density is often used to compare different energy storage technologies. This parameter relates the storage capacity to the size or the mass of the system, essentially showing how much energy (Wh) can be stored per unit cell, unit mass (kg), or unit volume (liter) of the material or device.
For example, energy densities for different types of batteries are listed in the table below [IES, 2011]:
Of course, we are interested to store as much energy as possible while using as small and light device as possible for this purpose. From the table above we can conclude, for example, that a fully charged Lead-Acid battery will run out of charge much sooner than a fully charged Li-ion battery of the same mass/size.
Energy density is related to capacity and determines the duration of power generation. Also materials with higher energy density help make the power block more compact, which is useful in portable electronics and vehicle applications.
Just for comparison, the energy density of the pumped hydro storage is 0.2—2 Wh/kg, which is rather low and requires significant masses of water and large reservoir size to deliver utility scale power.
Check Your Understanding Question 3 (Multiple Choice)
Power density.
Power density (measured in W/kg or W/liter) indicates how quickly a particular storage system can release power. Storage devices with higher power density can power bigger loads and appliances without going oversize. Imagine an electric vehicle accelerating from 0 to 60 MPH – which takes a lot of power. If you look at the table below, you will see why Li-ion battery remains the technology of choice for powering electric vehicles, even though some other battery types exhibit similar energy densities.
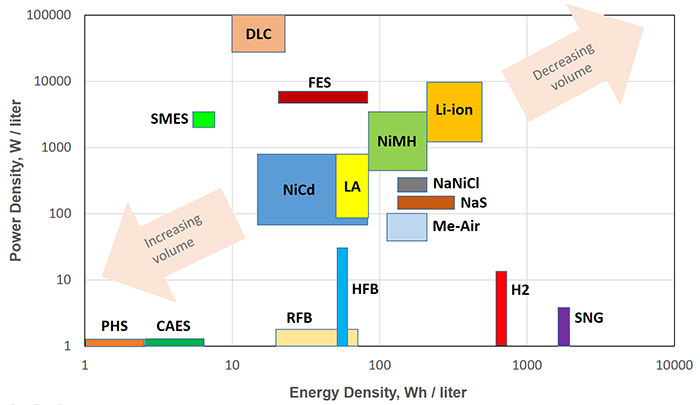
The technologies located in the lower left corner of the diagram (low energy density and low power density) take significant amount of space and material to enable the storage conversion and are mostly suitable for very large scale projects. Systems such as PHS and CAES also rely on the availability of specific landscape and geological features to accommodate the storage reservoirs.
The technologies located in the upper right corner of the diagram are most coveted for portable and efficient power supply, such as electric vehicles. These compact systems can carry a significant amount of energy and release it quickly on demand.
The technologies in the upper left corner are special devices that can be used in quick response electronics. These systems store small amounts of energy (and therefore charging can be fast), but are able to provide high power by releasing energy within short period of time.
Finally, the technologies in the lower right corner are characterized by slow charge and discharge, but the advantage is the total high amount of energy they are able to store, providing longer duration of energy supply.
Check Your Understanding Questions 4 & 5 (Multiple Choice)
Storage efficiency.
The main function of any storage device is to uptake and release power on demand. In case of a battery, for example, it would be electrochemical charge/discharge cycle; in case of pumped hydro storage, this process involves pumping water into the elevated reservoir and later releasing the flow through the turbine. Both charge and discharge processes include one or more energy conversions (Figure 10.3). In the figure, each arrow indicates the energy conversion from one form to another.
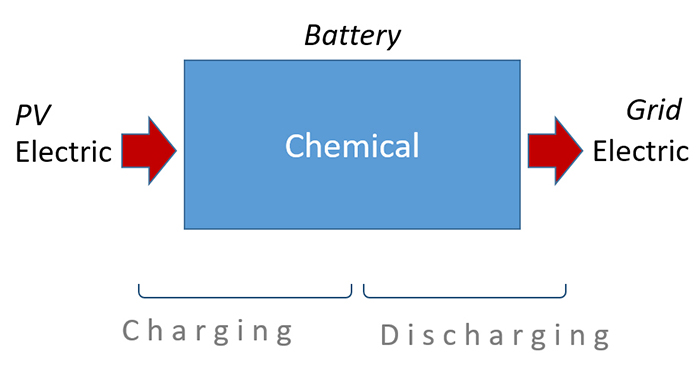
Regardless the number of transformations, the energy comes to its initial electric form, which is finally ready to be dispatched into the grid. This is the charge-discharge cycle, the "round trip".
In each conversion, energy is partially lost from the cycle and dissipated into the surroundings, and the efficiency of conversion at every step accounts for those losses.
Efficiencies of all energy conversion steps in this cycle are combined in the metric called round-trip efficiency , which essentially indicates the percentage of energy delivered by the storage system compared to the energy initially supplied to the storage system. The obvious goal is to minimize the conversion losses and thus maximize the overall storage efficiency.
Here are some round-trip efficiencies of various energy storage systems:
These numbers mean the following. For example, out of 1 MWh of energy spent to pump water up to the hydro storage, only 0.7-0.8 MWh will be available to use after the water is released to run the turbine and generator to produce electric power. The other 0.2-0.3 MWh of energy will be converted into non-useful forms of energy and “lost” from the cycle. Some of the energy losses occur in the auxiliary devices used in the energy storage process, very often in the form of waste heat. Furthermore, energy losses may be linked to the mechanical or material losses: for example, leaks and evaporation of water from pumped storage, air leaks in CAES, chemical degradation and incomplete reactions in batteries.
Check Your Understanding Questions 6 & 7 (Multiple Choice)
You are using an outdated browser. Please upgrade your browser to improve your experience.
- Resource Categorization
- Scenario Descriptions
- Representative Technology
- Methodology
Capacity Factor
Battery storage.
The ATB represents cost and performance for battery storage in the form of a 4-hour, utility-scale, lithium-ion battery system with a 15-year assumed life.
NREL has completed an analysis of the costs related to other battery sizes (4-hour to 0.5-hour) for utility-scale plants (Fu et al., 2018) Fu, Ran, Remo, Timothy W., & Margolis, Robert M. (2018). 2018 U.S. Utility-Scale Photovoltaics-Plus-Energy Storage System Costs Benchmark. (No. NREL/TP-6A20-71714). National Renewable Energy Laboratory. https://www.nrel.gov/docs/fy19osti/71714.pdf Click to jump to reference. ">(Fu et al., 2018) ; those costs are represented in the following figure from the report of that analysis.
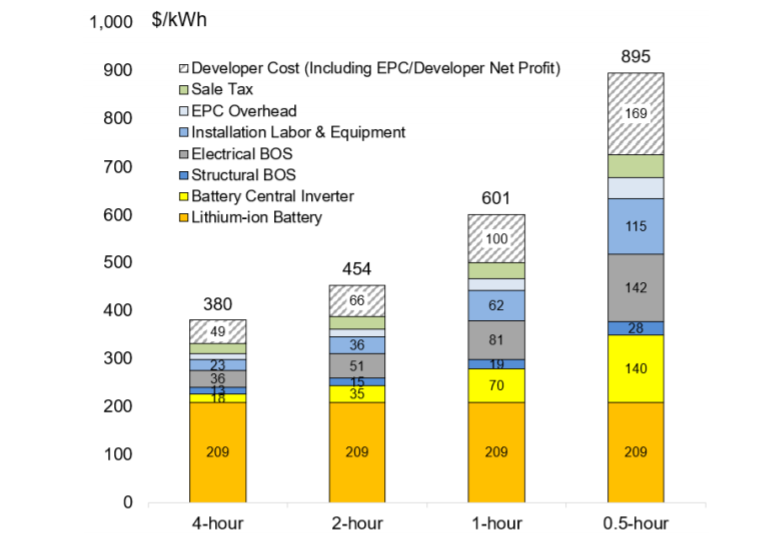
The ATB does not currently have costs for distributed battery storage, including costs for (1) behind-the-meter residential or commercial applications and (2) micro-grid or off-grid applications. Analysis by NREL of a residential battery-plus-solar PV system has resulted in a range of costs for such systems (Ardani et al., 2017) Ardani, Kristen, O'Shaughnessy, Eric, Fu, Ran, McClurg, Chris, Huneycutt, Joshua, & Margolis, Robert. (2017). Installed Cost Benchmarks and Deployment Barriers for Residential Solar Photovoltaics with Energy Storage: Q1 2016. (No. NREL/TP-7A40-67474). National Renewable Energy Laboratory. https://doi.org/10.2172/1338670 Click to jump to reference. ">(Ardani et al., 2017) .
Battery cost and performance projections in the 2020 ATB are based on a literature review of 19 sources published in 2018 or 2019, as described by Cole and Frazier (2020) Cole, Wesley, & Frazier, Will A. (2020). Cost Projections for Utility-Scale Battery Storage: 2020 Update. (No. NREL/TP-6A20-75385). National Renewable Energy Laboratory. https://www.nrel.gov/docs/fy20osti/75385.pdf Click to jump to reference. ">(2020) . Three projections from 2017 to 2050 are developed for scenario modeling based on this literature:
- Conservative Technology Innovation Scenario (Conservative Scenario): generally based on the maximum of literature projections of future CAPEX and O&M technology pathway analysis
- Moderate Technology Innovation Scenario (Moderate Scenario): generally based on the median of literature projections of future CAPEX and O&M technology pathway analysis
- Advanced Technology Innovation Scenario (Advanced Scenario): generally based on the low bound of literature projections of future CAPEX and O&M technology pathway analysis.
Capital Expenditures (CAPEX)
Definition: The literature review provided by Cole and Frazier (2020) Cole, Wesley, & Frazier, Will A. (2020). Cost Projections for Utility-Scale Battery Storage: 2020 Update. (No. NREL/TP-6A20-75385). National Renewable Energy Laboratory. https://www.nrel.gov/docs/fy20osti/75385.pdf Click to jump to reference. ">(2020) does not enumerate elements of the capital cost of lithium-ion batteries. However, the NREL storage cost report (Fu et al., 2018) Fu, Ran, Remo, Timothy W., & Margolis, Robert M. (2018). 2018 U.S. Utility-Scale Photovoltaics-Plus-Energy Storage System Costs Benchmark. (No. NREL/TP-6A20-71714). National Renewable Energy Laboratory. https://www.nrel.gov/docs/fy19osti/71714.pdf Click to jump to reference. ">(Fu et al., 2018) does detail a breakdown of capital costs with the actual battery pack being the largest component, but significant other costs are included. This breakdown is different if the battery is part of a hybrid system with solar PV. These relative costs for utility-scale standalone battery and battery + PV are demonstrated in the figure below.
Base Year : The Base Year cost estimate is taken from Feldman et al. (Forthcoming) Feldman, David, Vignesh Ramasamy, Ran Fu, Ashwin Ramdas, Jal Desai, and Robert Margolis. (Forthcoming). U.S. Solar Photovoltaic System and Energy Storage Cost Benchmark: Q1 2020. Golden, CO: National Renewable Energy Laboratory. Click to jump to reference. ">(Forthcoming) . Comparisons to other reported costs for 2018 and 2019 are included in Cole and Frazier (2020) Cole, Wesley, & Frazier, Will A. (2020). Cost Projections for Utility-Scale Battery Storage: 2020 Update. (No. NREL/TP-6A20-75385). National Renewable Energy Laboratory. https://www.nrel.gov/docs/fy20osti/75385.pdf Click to jump to reference. ">(2020) .
Within the ATB Data spreadsheet, costs are separated into energy and power cost estimates, which allow capital costs to be constructed for durations other than four hours according to the following equation:
For more information on the power versus energy cost breakdown, see Cole and Frazier (2020) Cole, Wesley, & Frazier, Will A. (2020). Cost Projections for Utility-Scale Battery Storage: 2020 Update. (No. NREL/TP-6A20-75385). National Renewable Energy Laboratory. https://www.nrel.gov/docs/fy20osti/75385.pdf Click to jump to reference. ">(2020) .
Future Projections : Future projections are taken from Cole and Frazier (2020) Cole, Wesley, & Frazier, Will A. (2020). Cost Projections for Utility-Scale Battery Storage: 2020 Update. (No. NREL/TP-6A20-75385). National Renewable Energy Laboratory. https://www.nrel.gov/docs/fy20osti/75385.pdf Click to jump to reference. ">(2020) , which generally used the median of published cost estimates to develop a Mid Technology Cost Scenario and the minimum values to develop a Low Technology Cost Scenario. Analysts' judgment is used to select the long-term projections to 2050 from a sparse data set.
Operation and Maintenance (O&M) Costs
Base Year : Cole and Frazier (2020) Cole, Wesley, & Frazier, Will A. (2020). Cost Projections for Utility-Scale Battery Storage: 2020 Update. (No. NREL/TP-6A20-75385). National Renewable Energy Laboratory. https://www.nrel.gov/docs/fy20osti/75385.pdf Click to jump to reference. ">(2020) assumed no variable operation and maintenance (VOM) cost. All operating costs are instead represented using fixed operation and maintenance (FOM) costs. The FOM costs include augmentation costs needed to keep the battery system operating at rated capacity for its lifetime. In the 2020 ATB, FOM is defined as the value needed to compensate for degradation to enable the battery system to have a constant capacity throughout its life. The literature review (Cole & Frazier, 2020) Cole, Wesley, & Frazier, Will A. (2020). Cost Projections for Utility-Scale Battery Storage: 2020 Update. (No. NREL/TP-6A20-75385). National Renewable Energy Laboratory. https://www.nrel.gov/docs/fy20osti/75385.pdf Click to jump to reference. ">(Cole & Frazier, 2020) states that FOM costs are estimated at 2.5% of the capital costs in dollars per kilowatt.
Future Years : In the 2020 ATB, the FOM cost remains constant at 2.5% of capital costs in all scenarios.
The cost and performance of the battery systems are based on an assumption of approximately one cycle per day. Therefore, a 4-hour device has an expected capacity factor of 16.7% (4/24 = 0.167), and a 2-hour device has an expected capacity factor of 8.3% (2/24 = 0.083). Because FOM includes costs to keep the system at rated capacity, no degradation is assumed.
Round-Trip Efficiency
Round-trip efficiency is the ratio of useful energy output to useful energy input. Cole and Frazier (2020) Cole, Wesley, & Frazier, Will A. (2020). Cost Projections for Utility-Scale Battery Storage: 2020 Update. (No. NREL/TP-6A20-75385). National Renewable Energy Laboratory. https://www.nrel.gov/docs/fy20osti/75385.pdf Click to jump to reference. ">(2020) identified 85% as a representative round-trip efficiency, and the 2020 ATB adopts this value.
The following references are specific to this page; for all references in this ATB, see References .
Ardani, Kristen, O'Shaughnessy, Eric, Fu, Ran, McClurg, Chris, Huneycutt, Joshua, & Margolis, Robert. (2017). Installed Cost Benchmarks and Deployment Barriers for Residential Solar Photovoltaics with Energy Storage: Q1 2016. (No. NREL/TP-7A40-67474). National Renewable Energy Laboratory. https://doi.org/10.2172/1338670
Cole, Wesley, & Frazier, Will A. (2020). Cost Projections for Utility-Scale Battery Storage: 2020 Update. (No. NREL/TP-6A20-75385). National Renewable Energy Laboratory. https://www.nrel.gov/docs/fy20osti/75385.pdf
Feldman, David, Vignesh Ramasamy, Ran Fu, Ashwin Ramdas, Jal Desai, and Robert Margolis. (Forthcoming). U.S. Solar Photovoltaic System and Energy Storage Cost Benchmark: Q1 2020. Golden, CO: National Renewable Energy Laboratory.
Fu, Ran, Remo, Timothy W., & Margolis, Robert M. (2018). 2018 U.S. Utility-Scale Photovoltaics-Plus-Energy Storage System Costs Benchmark. (No. NREL/TP-6A20-71714). National Renewable Energy Laboratory. https://www.nrel.gov/docs/fy19osti/71714.pdf
Developed with funding from the U.S. Department of Energy’s Office of Energy Efficiency and Renewable Energy.
Experimental Analysis of Efficiencies of a Large Scale Energy Storage System
Ieee account.
- Change Username/Password
- Update Address
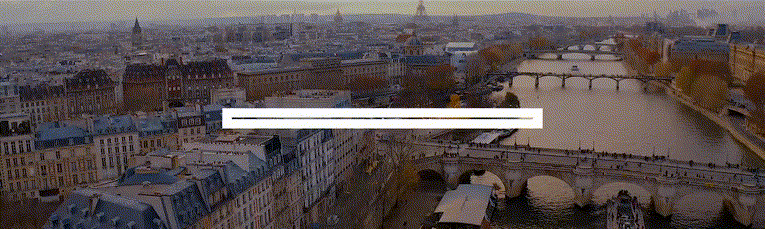
Purchase Details
- Payment Options
- Order History
- View Purchased Documents
Profile Information
- Communications Preferences
- Profession and Education
- Technical Interests
- US & Canada: +1 800 678 4333
- Worldwide: +1 732 981 0060
- Contact & Support
- About IEEE Xplore
- Accessibility
- Terms of Use
- Nondiscrimination Policy
- Privacy & Opting Out of Cookies
A not-for-profit organization, IEEE is the world's largest technical professional organization dedicated to advancing technology for the benefit of humanity. © Copyright 2024 IEEE - All rights reserved. Use of this web site signifies your agreement to the terms and conditions.
- Visit the GivEnergy cloud

- All in One – battery plus inverter
- AC coupled inverter
- Hybrid inverter
- Battery storage
- Full energy ecosystem overview
- Find an installer
- Power conversion system (PCS)
- Battery packs
- Commercial battery rack
- SME battery system
- Battery storage container
Energy management software
- GivEnergy app
- GivEnergy portal
- Home battery storage
- Commercial battery storage
- Sustainable construction projects
- Wholesalers
- Energy suppliers – E.ON partner
- Energy suppliers – Octopus
- Energy aggregators
- Solar industry – Open Solar
- Solar industry – Easy PV
- Giv-Gallery
- Case studies
- Support hall of fame
- Why choose GivEnergy?
- Refer a friend
- Find a distributor
- Knowledge base
- Community forum
Documentation
- Resource hub
- Product brochure 2024
- GivEnergy API
- Server status
- View all posts
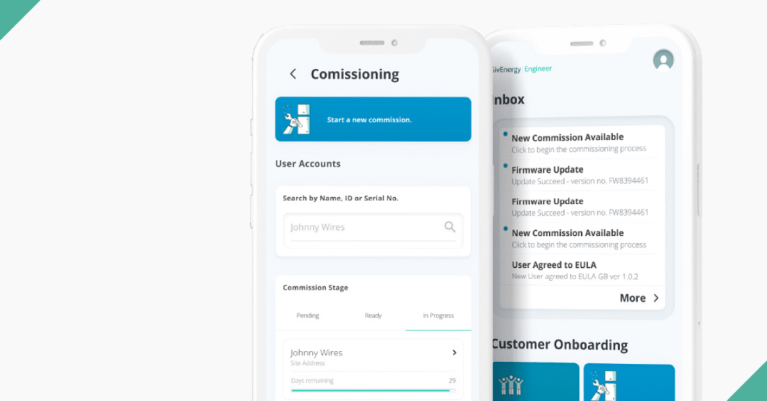
Sneak preview: the new GivEngineer app
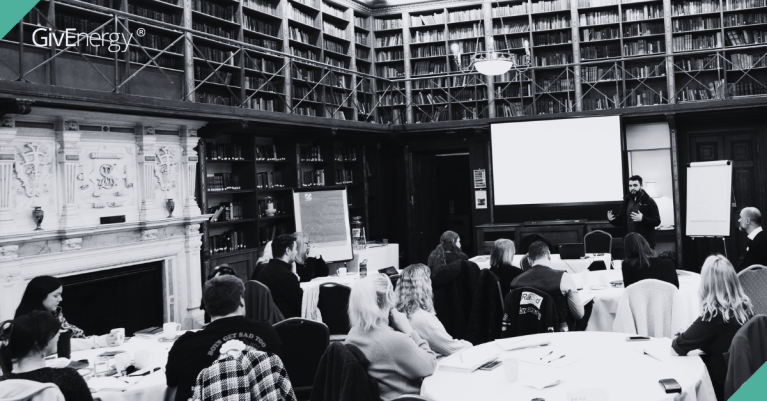
Keele University and GivEducation partner to deliver a first-of-its-kind edtech...
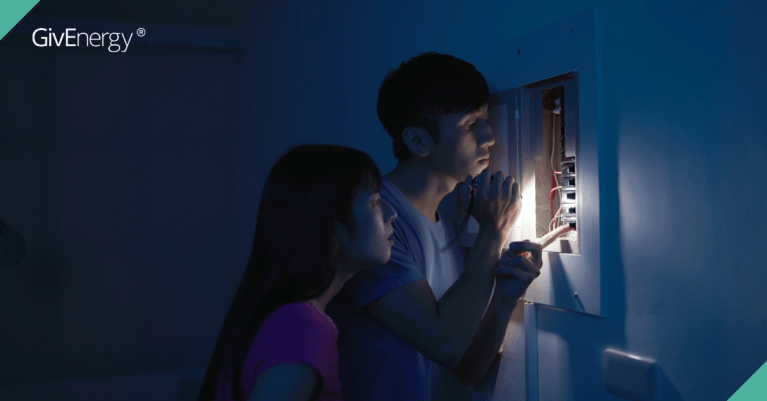
Home backup power: why solar panels alone aren’t enough
- FIND AN INSTALLER
- Home-owners
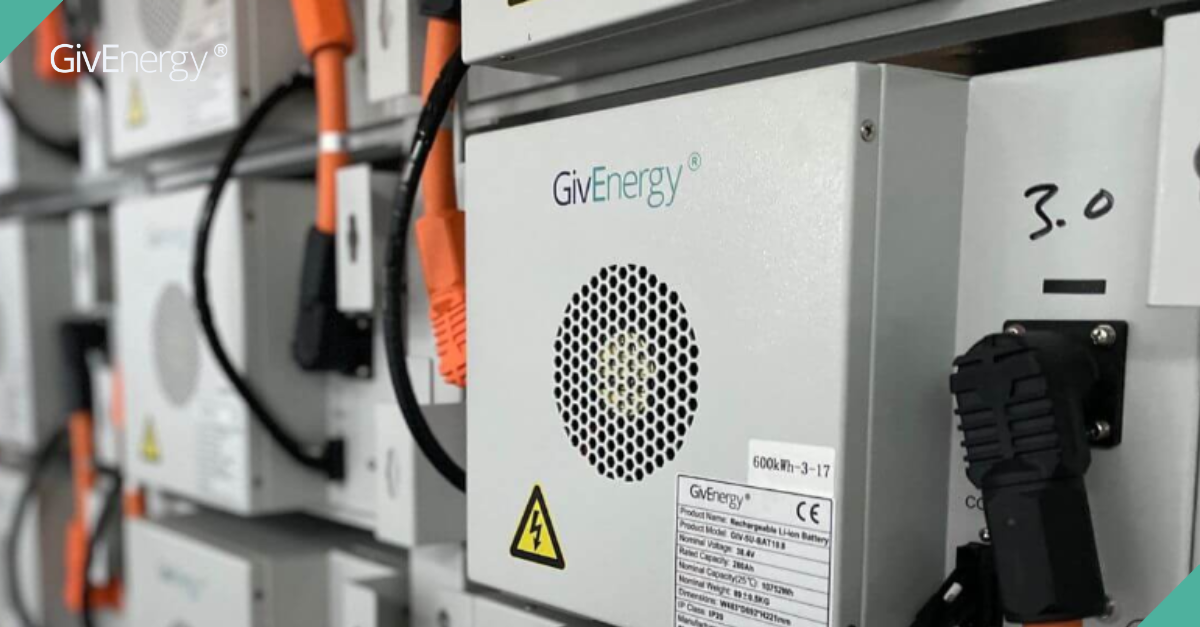
- January 30, 2024
- Blog , Commercial battery storage , Domestic battery storage , Explain like I’m 5
What is round trip efficiency in battery storage?
Round trip efficiency (RTE) is a measure of how efficiently a battery can store and discharge energy. Find out why it’s crucial in the world of BESS.
Terminology in the world of battery storage can seem daunting to the average Joe.
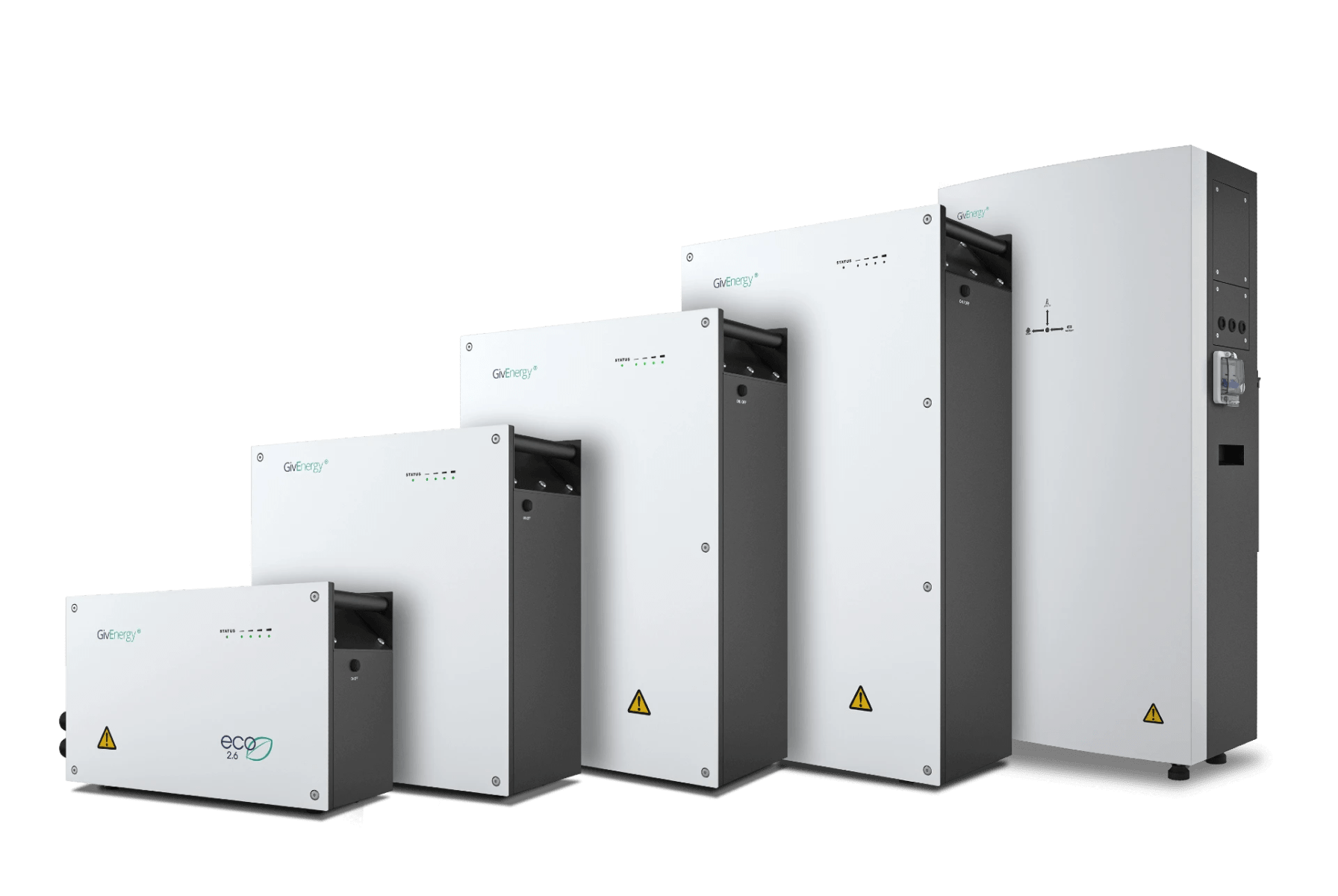
Luckily, we’re here to help.
Round trip efficiency (RTE) is something you may have come across in relation to batteries.
In a nutshell, RTE measures how efficiently a battery can store and discharge energy.
How is RTE calculated? Why are there no batteries with 100% RTE? How has RTE in storage batteries improved in recent years?
Read on to find the answers to these questions.
How to calculate the RTE of a battery?
The RTE of a battery can be calculated as a percentage using a simple formula shown below:
Energy output ÷ energy input x 100 = RTE
Let’s demonstrate what this means in practical terms with an example.
Mrs Jones installs a storage battery for her home . As she and her family typically use 10 kWh of electricity per day, she opts for a 10 kWh storage battery.
As someone who is both eco-conscious and has an above-average income, Mrs Jones installs both solar panels and a wind turbine to power her battery storage system. This means she can charge her 10 kWh battery from renewable sources.

However, Mrs Jones soon realises that she isn’t getting 10 kWh per day from her storage battery.
In reality, she gets 8 kWh per day. That’s because her 10 kWh battery has 80% round trip efficiency.
If she had opted for a 10 kWh battery with 90% round trip efficiency, she would get… yep… 9 kWh per day.
For her remaining 2 kWh per day of electricity needs, she draws energy from the grid.
Why do batteries lose energy?
Unfortunately, batteries always lose a small amount of energy during operation.
This is usually because of heat loss, self-discharge, high internal cell resistance or other factors.
Are there any batteries with 100% RTE?
You can think of it like this: if anyone ever tries to sell you a 100% RTE battery, run a mile because they’re almost certainly lying.
What is the RTE of a typical battery?
RTE varies among different types of storage batteries .

For older battery systems, 80% round trip efficiency would have been considered a good standard. Some evidence suggests the typical lithium-ion battery – a popular choice for modern battery energy storage systems and electric vehicles – has round trip efficiency of around 83%.
GivEnergy’s own batteries – using LiFePO4 (lithium iron phosphate) – have achieved 93% round trip efficiency .
RTE – not to be ignored
Grid-level battery storage is becoming increasingly common to accommodate the growth in renewables, especially solar and wind.
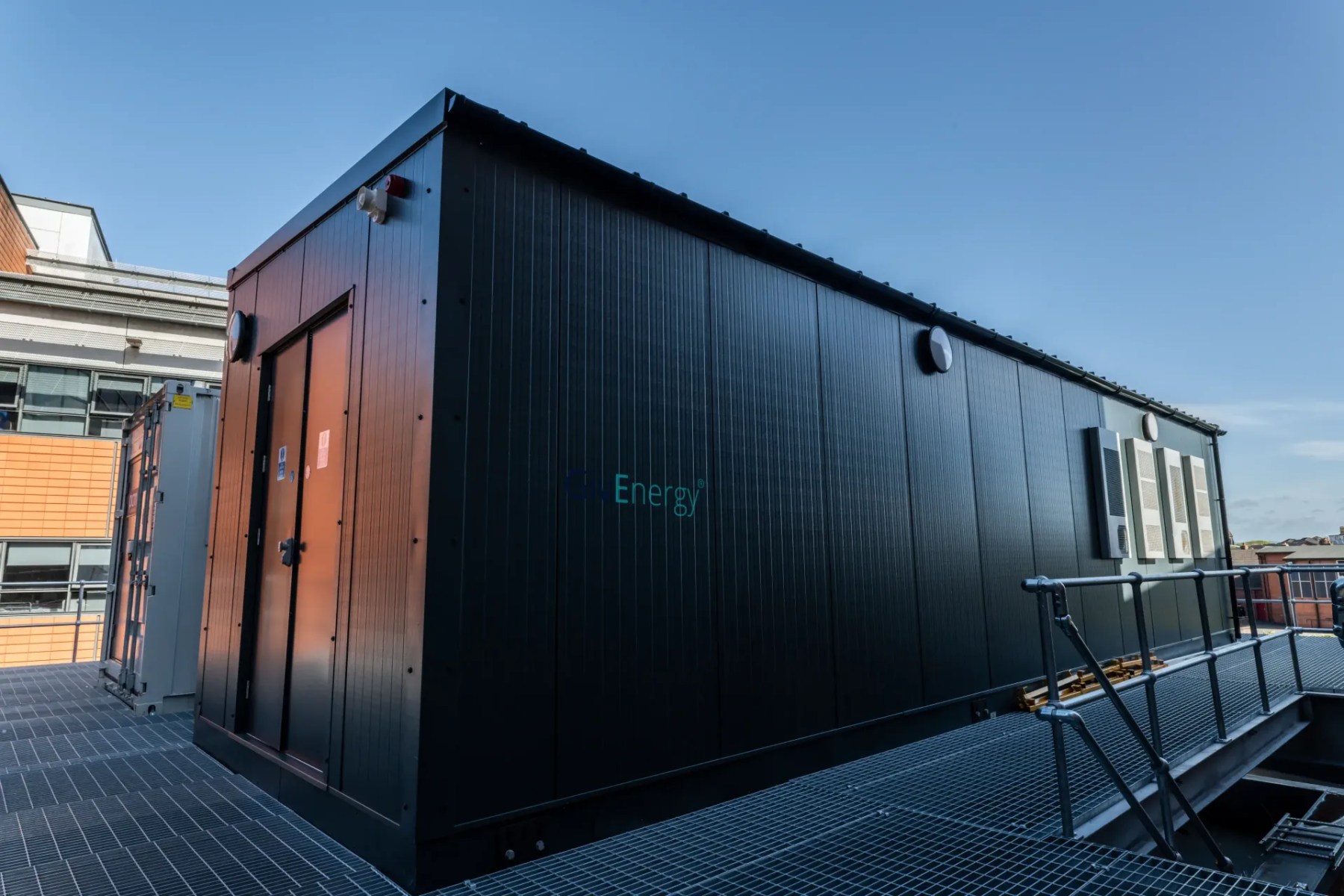
Round trip efficiency is a factor that decision-makers need to take into account when assessing the overall efficiency of an energy storage system.
And it’s something YOU also need to bear in mind when installing your own battery storage system for your home or business.
Remember: 100% round trip efficiency is a lie! However, 93% round trip efficiency with a GivEnergy battery using LiFePO4 technology is not a lie.
Looking to play your part in the energy storage revolution? Get started by finding an approved GivEnergy installer today.
Further reading
- Explain like I’m 5: depth of discharge
- LiFePO4 battery: what is it & why is it best for BESS?
- The superior choice: embracing LiFePO4 batteries over NMC technology
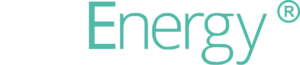
Quick Links
Getting started with...
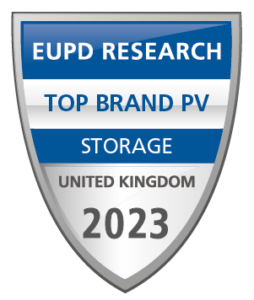
Product images used are for illustrative purposes. The finished setup will vary from installation to installation, and will include all your needed cable connections, metering, any (outdoor) canopies, etc.
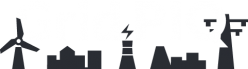
GridProjectIQ Documentation
Energy Storage System Efficiency
The round trip efficiency (RTE) of an energy storage system is defined as the ratio of the total energy output by the system to the total energy input to the system, as measured at the point of connection. The RTE varies widely for different storage technologies. A high value means that the incurred losses are low.
Reference Information
The typical RTE values for different technologies along with the source of information are provided below. If the reference provides a range of values instead of a single value, the median value of the range has been used.
- Lithium-ion (83%) : W. G. Manuel, “Energy Storage Study 2014”, 2014.
- Vanadium redox flow (75%) : V. Viswanathan, M. Kintner-Meyer, P. Balducci and C. Jin, “National Assessment of Energy Storage for Grid Balancing and Arbitrage, Phase II, Volume 2: Cost and Performance Characterization”, Sept. 2013.
- Sodium sulfur (75%) : W. G. Manuel, “Energy Storage Study 2014”, 2014.
- Advanced lead-acid (85%) : IEC, “Electrical Energy Storage: White Paper”, 2011.
- Flywheel (81%) : V. Viswanathan, M. Kintner-Meyer, P. Balducci and C. Jin, “National Assessment of Energy Storage for Grid Balancing and Arbitrage, Phase II, Volume 2: Cost and Performance Characterization”, Sept. 2013.
- Compressed air (50%) : IEC, “Electrical Energy Storage: White Paper”, 2011.
- Pumped hydro (81%) : V. Viswanathan, M. Kintner-Meyer, P. Balducci and C. Jin, “National Assessment of Energy Storage for Grid Balancing and Arbitrage, Phase II, Volume 2: Cost and Performance Characterization”, Sept. 2013.
Pumped Storage Hydropower
The 2022 ATB data for pumped storage hydropower (PSH) are shown above. Base Year capital costs and resource characterizations are taken from a national closed-loop PSH resource assessment completed under the U.S. Department of Energy (DOE) HydroWIRES Project D1: Improving Hydropower and PSH Representations in Capacity Expansion Models. Resource assessment and cost assumptions are documented by (Rosenlieb et al., 2022) . This effort considered only closed-loop systems due to their relatively lower environmental impacts, so open-loop and other configurations are not included in these estimates. Operation and maintenance O&M costs and round-trip efficiency are based on estimates for a 1,000-MW system reported in the 2020 DOE Grid Energy Storage Technology Cost and Performance Assessment . (Mongird et al., 2020) . Projected changes in capital costs are based on the DOE Hydropower Vision study (DOE, 2016) and assume different degrees of technology improvement and technological learning.
The three scenarios for technology innovation are:
- Conservative Technology Innovation Scenario (Conservative Scenario): no change from baseline CAPEX and O&M costs through 2050
- Moderate Technology Innovation Scenario (Moderate Scenario): no change from baseline CAPEX and O&M costs through 2050, consistent with the Reference case in the DOE Hydropower Vision study (DOE, 2016)
- Advanced Technology Innovation Scenario (Advanced Scenario): CAPEX reductions of 12% by 2050 based on improved process and design improvements along with advanced manufacturing, new materials, and other technology improvements, consistent with Advanced Technology in the DOE Hydropower Vision study (DOE, 2016) ; no changes to O&M.
Resource Categorization
Resource categorization from a national closed-loop PSH resource assessment is described in detail by (Rosenlieb et al., 2022) . Individual sites are identified using geospatial algorithms to delineate potential reservoir boundaries, exclude reservoirs that violate technical potential criteria (e.g., protected land, critical habitat), find all possible reservoir pairings, and then eliminate overlapping reservoirs to produce the least-cost set of non-overlapping reservoir pairs. Underlying data are site-specific, but for the ATB, resource classes are binned by capital cost such that each class contains a roughly equal amount of total national PSH capacity potential. Binning is done at the national level for the data tables below, and other representations use region-specific cost bins to better represent the distribution of site characteristics in each region. Physical characteristics and capital cost statistics for each ATB class are included in the table below.
Resource Class Capacity and Capital Costs
Resource Class Design Values
Scenario Descriptions
Cost reductions in the Advanced Scenario reflect various types of technology innovations that could be applied to PSH facilities. These potential innovations, which are discussed in the DOE Hydropower Vision Roadmap (DOE, 2016) , are largely similar to technology pathways for hydropower without pumping.
Summary of Technology Innovation: Advanced Scenario
Representative Technology
The resource assessment procedure requires several design specifications to be defined up front, and for the resource included in the ATB, these include a fixed 30-m dam height, a minimum 300-m hydraulic head height, and a maximum reservoir distance of 15 times the head height (Rosenlieb et al., 2022) . Upper and lower reservoir volumes are also assumed to be within 20% of each other. Given the resulting technical specifications of each reservoir pair, the powerhouse (turbine, generator, and electrical equipment) can be sized flexibly for a given reservoir pair, and here all data assume the powerhouse is sized for exactly 10 hours of storage duration (i.e., a maximum of 10 hours generating at rated capacity).
Methodology
This section describes the methodology to develop assumptions for CAPEX, O&M, and round-trip efficiency.
Capital Expenditures (CAPEX)
Capital costs are first calculated for each site using the PSH cost model from Australia National University (Andrew Blakers et al., 2019) adjusted to use a 33% project contingency factor instead of the base 20% assumption to better align with other technologies and U.S. industry practice. The cost model uses reservoir and powerhouse characteristics as inputs to generalized equations for PSH overnight capital cost. These raw costs are then further calibrated to more closely match hydropower industry expectations by multiplying site costs by a factor equal to the ratio of the central CAPEX estimate in (Mongird et al., 2020) for a 1,000-MW, 10-hour facility to the median CAPEX of all sites in the capacity range of 900–1,100 MW (Mongird et al., 2020) . This factor is equal to 1.51, and due to the limited amount of available cost data, this factor is applied uniformly to all sites. Grid connection costs are then added based on the distance from the powerhouse location (assumed at the lower reservoir) to the nearest high-voltage transmission line node (Maclaurin et al., 2021) . Cost assessment is described in greater detail in (Rosenlieb et al., 2022) .
The maps below plot median CAPEX in each state for each of 15 resource classes when individual sites are binned by cost separately for each state. Some states have zero sites identified, largely due to insufficient elevation differences to meet a 300 m minimum head height criteria. The ratio of distance between reservoirs to head height (L/H ratio) is also shown for individual sites. The display also includes links to a bar chart and a tabular display. The bar chart shows more granular data for each balancing area defined in the Regional Energy Deployment System ( ReEDS ) capacity expansion model (Ho et al., 2021) along with the state average PSH capital cost. The table allows the data to be filtered by class and balancing area to view region- or class-specific data.
Regional PSH Capital Cost by Class
Operation and Maintenance (O&M) Costs
(Mongird et al., 2020) characterize PSH O&M costs using a literature review of recently published sources of PSH cost and performance data. For the 2022 ATB, we use cost estimates for a 1,000-MW plant, which has lower labor costs per power output capacity than a smaller facility. O&M costs also include component costs for standard maintenance, refurbishment, and repair. O&M cost reductions are not projected because the relevant technical components are assumed to be mature, so they are constant and identical across all scenarios.
Round-Trip Efficiency
Round-trip efficiency is also based on a literature review by (Mongird et al., 2020) , who report a range of 70%–87% across several sources. The value of 80% is taken as a central estimate, and no improvements are projected either in (Mongird et al., 2020) or here because the relevant technical components are assumed to be mature. Thus, round-trip efficiency is constant and identical across all scenarios.
The following references are specific to this page; for all references in this ATB, see References .
Rosenlieb, Evan, Donna Heimiller, and Stuart Cohen. “Closed-Loop Pumped Storage Hydropower Resource Assessment for the United States.” Golden, CO: National Renewable Energy Laboratory, 2022. https://www.nrel.gov/docs/fy22osti/81277.pdf .
Mongird, Kendall, Vilayanur Viswanathan, Jan Alam, Charlie Vartanian, Vincent Sprenkle, and Richard Baxter. “2020 Grid Energy Storage Technology Cost and Performance Assessment.” Washington, D.C.: U. S. Department of Energy, December 2020. https://www.energy.gov/energy-storage-grand-challenge/downloads/2020-grid-energy-storage-technology-cost-and-performance .
DOE. “Hydropower Vision: A New Chapter for America’s Renewable Electricity Source.” Washington, D.C.: U.S. Department of Energy, 2016. https://doi.org/10.2172/1502612 .
Maclaurin, Galen, Nicholas Grue, Anthony Lopez, Donna Heimiller, Michael Rossol, Grant Buster, and Travis Williams. “The Renewable Energy Potential (ReV) Model: A Geospatial Platform for Technical Potential and Supply Curve Modeling.” Golden, CO: National Renewable Energy Laboratory, 2021. https://doi.org/10.2172/1563140 .
Ho, Jonathan, Jonathon Becker, Maxwell Brown, Patrick Brown, Ilya (ORCID:0000000284917814) Chernyakhovskiy, Stuart Cohen, Wesley (ORCID:000000029194065X) Cole, et al. “Regional Energy Deployment System (ReEDS) Model Documentation: Version 2020.” Golden, CO: National Renewable Energy Laboratory, June 9, 2021. https://doi.org/10.2172/1788425 .
Andrew Blakers, Matthew Stocks, Bin Lu, Kirsten Anderson, and Anna Nadolny. “Global Pumped Hydro Atlas.” Australian National University, 2019. http://re100.eng.anu.edu.au/research/phes /.
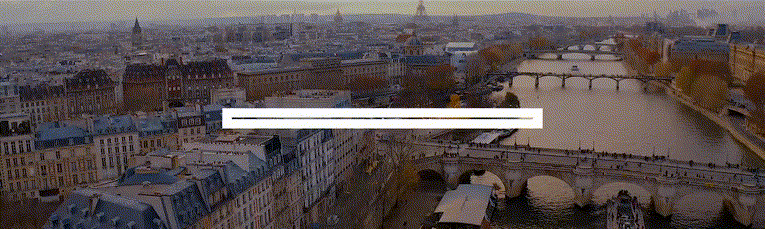
COMMENTS
The round trip efficiency (RTE), also known as AC/AC efficiency, refers to the ratio between the energy supplied to the storage system (measured in MWh) and the energy retrieved from it (also measured in MWh). This efficiency is expressed as a percentage (%). The round trip efficiency is a crucial factor in determining the effectiveness of ...
Round-Trip Efficiency. Round-trip efficiency is the ratio of useful energy output to useful energy input. (Mongird et al., 2020) identified 86% as a representative round-trip efficiency, and the 2022 ATB adopts this value. In the same report, testing showed 83-87%, literature range of 77-98%, and a projected increase to 88% in 2030.
Round-trip efficiency is a measure of the amount of energy put into a system compared to the amount dispatched, and is expressed as a percentage. A system with a high RTE (75%+) is able to ...
• Round-trip efficiency, measured as a percentage, is a ratio of the energy charged to the battery to the energy discharged from the battery. It can represent the total DC-DC or AC-AC efficiency of the battery system, including losses from self-discharge and other electrical losses. Although battery manufacturers often refer to the
Round-trip efficiency is the percentage of electricity put into storage that is later retrieved. The higher the round-trip efficiency, the less energy is lost in the storage process. According to data from the U.S. Energy Information Administration (EIA), in 2019, the U.S. utility-scale battery fleet operated with an average monthly round-trip ...
The round-trip efficiency ranges generally between 70 % and 80 %. It takes values inferior to 100 % due to the different losses occurring within the system. The objective of this study is to assess the round-trip efficiency of GES system by evaluating the different factors that cause energy losses during the system's operation.
Round-Trip Efficiency. Round-trip efficiency is the ratio of useful energy output to useful energy input. Based on Cole et al. (Cole and Karmakar, 2023), the 2023 ATB assumes a round-trip efficiency of 85%. References . The following references are specific to this page; for all references in this ATB, see References.
The obvious goal is to minimize the conversion losses and thus maximize the overall storage efficiency. Here are some round-trip efficiencies of various energy storage systems: Table 10.5 Round-Trip Efficiencies of Various Energy Storage Systems; Storage system Round-trip efficiency, % Lead-Acid battery: 75-90: Li-ion battery: 85-98: Pumped ...
The system was designed to offer a nominal power size of 150 kW e and energy storage capacity of 600 kWh e for an 8-hour storage cycle. This work presents evidence of the system Round-trip efficiency (RTE), which is considered as a fundamental performance metric for large-scale energy storage technologies.
Degradation is a function of this usage rate of the model and systems might need to be replaced at some point during the analysis period. We use the capacity factor for a 4-hour device as the default value for ATB. Round-Trip Efficiency. Round-trip efficiency is the ratio of useful energy output to useful energy input.
Round-Trip Efficiency. Round-trip efficiency is the ratio of useful energy output to useful energy input. (Mongird et al., 2020) identified 86% as a representative round-trip efficiency, and the 2022 ATB adopts this value. In the same report, testing showed 83-87%, literature range of 77-98%, and a projected increase to 88% in 2030.
Round-trip efficiency and useable energy are exemplary performance and health metrics. To measure such system parameters in a controlled procedure, reference performance tests (RPT) are defined to be conducted intervals. To also at measure parameters during normal systems operation, real-time monitoring tests (RTM), which collect data during
Because FOM includes costs to keep the system at rated capacity, no degradation is assumed. Round-Trip Efficiency. Round-trip efficiency is the ratio of useful energy output to useful energy input. Cole and Frazier identified 85% as a representative round-trip efficiency, and the 2020 ATB adopts this value.
Recent times have witnessed significant progress in battery technology due to the growing demand for energy storage systems in various applications. Consequently, battery efficiency has become a crucial aspect of modern battery technology since it directly influences battery performance and lifespan. To guarantee the optimal performance and longevity of batteries, it is essential to measure ...
This paper documents the investigation into determining the round trip energy efficiency of a 2MW Lithium-titanate battery energy storage system based in Willenhall (UK). This research covers the battery and overall system efficiency as well as an assessment of the auxiliary power consumption of the system. The results of this analysis can be used to run the system at its optimal operating ...
RTE varies among different types of storage batteries. For older battery systems, 80% round trip efficiency would have been considered a good standard. Some evidence suggests the typical lithium-ion battery - a popular choice for modern battery energy storage systems and electric vehicles - has round trip efficiency of around 83%. GivEnergy ...
System round-trip efficiency. 1. Introduction. The problem of energy waste and environmental pollution caused by unreasonable energy structures are the inevitable consequences of the world's overdependence on coal-fired power generation, which restricts the development of primary energy.
The round trip efficiency (RTE) of an energy storage system is defined as the ratio of the total energy output by the system to the total energy input to the system, as measured at the point of connection. The RTE varies widely for different storage technologies. A high value means that the incurred losses are low.
The superior energy density renders hybrid Li-air batteries (HLABs) promising candidate energy storage systems to enhance the sustainability of power grids. Nevertheless, HLABs operated at ambient temperature struggle to meet power and cycle life performance requirements for commercial application. At the same time, low-grade heat is abundantly available from industrial processes as well as ...
Battery storage systems' round-trip efficiency ranges between 85% and 95%, but losses to heat and parasitic loads are the current hurdles. This hurts the site's energy usage. All the constraints of the ESS require ongoing technology advancements, optimization, and planning to ensure the demand can be accommodated by the equipment and the ...
The optimized PTLAES system can achieve lower LCOS than lithium-ion batteries when the discharge duration exceeds 3.5 h. Furthermore, it was shown that key criteria including round-trip efficiency, LCOS, and energy density can be traded off over a broad design space of different system configurations, parameter combinations, and material choices.
The paper has discussed round-trip efficiency (η round-trip) as the critical figure of merit to determine the viability of an energy storage system. The aim of the study is to offer a comprehensive analysis of the options available for each of the systems implied in a P2P-mGT, using round-trip efficiency as the figure of merit for comparison.
The 2021 ATB represents cost and performance for battery storage with two representative systems: a 3 kW / 6 kWh (2 hour) system and a 5 kW / 20 kWh (4 hour) system. It represents lithium-ion batteries only at this time. ... Round-Trip Efficiency. Round-trip efficiency is the ratio of useful energy output to useful energy input. (Mongird et al ...
Poliak argues that future audio setups will get OTA upgrades that can both improve sound quality and vehicle efficiency, and says the company's current hardware computing systems (dubbed "Neuron ...
This effort considered only closed-loop systems due to their relatively lower environmental impacts, so open-loop and other configurations are not included in these estimates. Operation and maintenance O&M costs and round-trip efficiency are based on estimates for a 1,000-MW system reported in the 2020 DOE Grid Energy Storage Technology Cost ...