
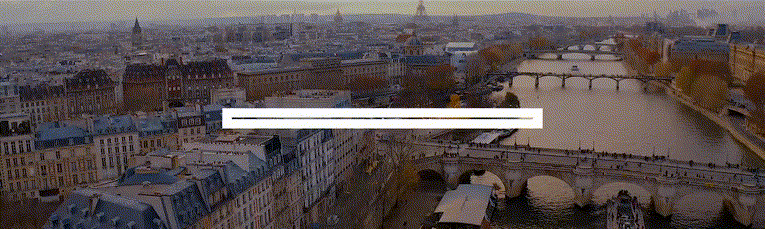
Anatomy of an Electromagnetic Wave
Energy, a measure of the ability to do work, comes in many forms and can transform from one type to another. Examples of stored or potential energy include batteries and water behind a dam. Objects in motion are examples of kinetic energy. Charged particles—such as electrons and protons—create electromagnetic fields when they move, and these fields transport the type of energy we call electromagnetic radiation, or light.
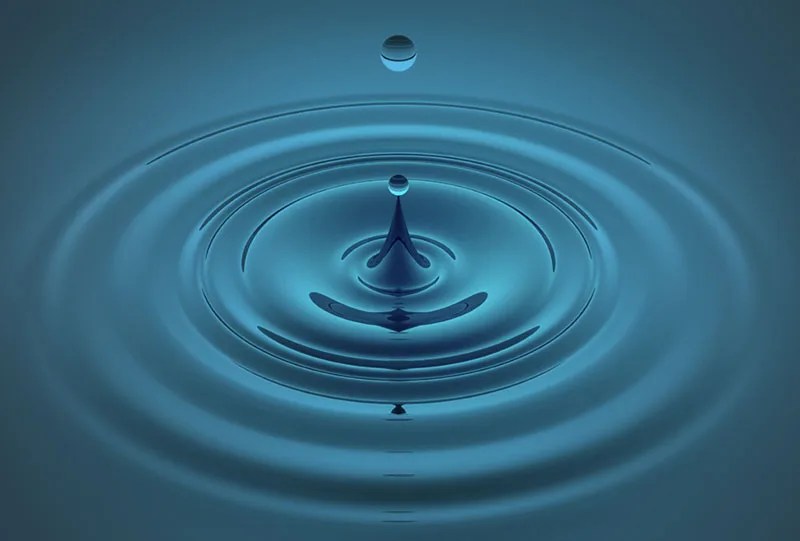
What are Electromagnetic and Mechanical waves?
Mechanical waves and electromagnetic waves are two important ways that energy is transported in the world around us. Waves in water and sound waves in air are two examples of mechanical waves. Mechanical waves are caused by a disturbance or vibration in matter, whether solid, gas, liquid, or plasma. Matter that waves are traveling through is called a medium. Water waves are formed by vibrations in a liquid and sound waves are formed by vibrations in a gas (air). These mechanical waves travel through a medium by causing the molecules to bump into each other, like falling dominoes transferring energy from one to the next. Sound waves cannot travel in the vacuum of space because there is no medium to transmit these mechanical waves.
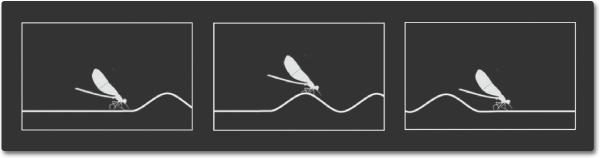
ELECTROMAGNETIC WAVES
Electricity can be static, like the energy that can make your hair stand on end. Magnetism can also be static, as it is in a refrigerator magnet. A changing magnetic field will induce a changing electric field and vice-versa—the two are linked. These changing fields form electromagnetic waves. Electromagnetic waves differ from mechanical waves in that they do not require a medium to propagate. This means that electromagnetic waves can travel not only through air and solid materials, but also through the vacuum of space.
In the 1860's and 1870's, a Scottish scientist named James Clerk Maxwell developed a scientific theory to explain electromagnetic waves. He noticed that electrical fields and magnetic fields can couple together to form electromagnetic waves. He summarized this relationship between electricity and magnetism into what are now referred to as "Maxwell's Equations."
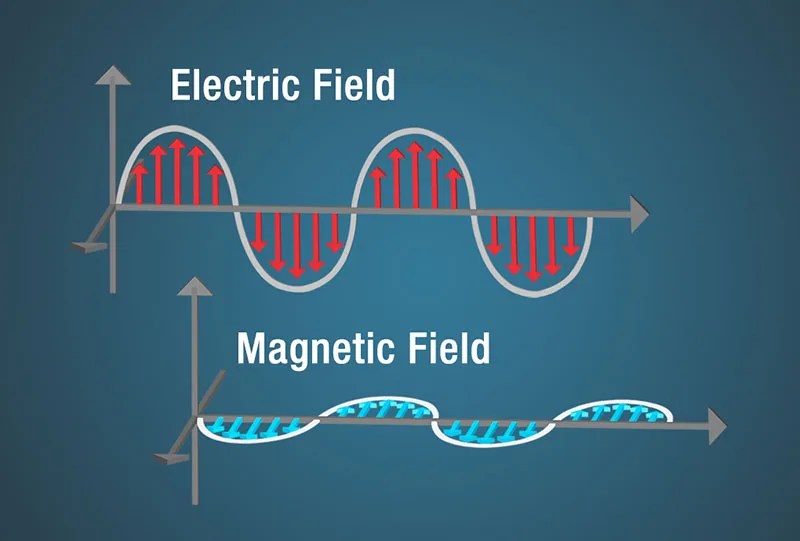
Heinrich Hertz, a German physicist, applied Maxwell's theories to the production and reception of radio waves. The unit of frequency of a radio wave -- one cycle per second -- is named the hertz, in honor of Heinrich Hertz.
His experiment with radio waves solved two problems. First, he had demonstrated in the concrete, what Maxwell had only theorized — that the velocity of radio waves was equal to the velocity of light! This proved that radio waves were a form of light! Second, Hertz found out how to make the electric and magnetic fields detach themselves from wires and go free as Maxwell's waves — electromagnetic waves.
WAVES OR PARTICLES? YES!
Light is made of discrete packets of energy called photons. Photons carry momentum, have no mass, and travel at the speed of light. All light has both particle-like and wave-like properties. How an instrument is designed to sense the light influences which of these properties are observed. An instrument that diffracts light into a spectrum for analysis is an example of observing the wave-like property of light. The particle-like nature of light is observed by detectors used in digital cameras—individual photons liberate electrons that are used for the detection and storage of the image data.
POLARIZATION
One of the physical properties of light is that it can be polarized. Polarization is a measurement of the electromagnetic field's alignment. In the figure above, the electric field (in red) is vertically polarized. Think of a throwing a Frisbee at a picket fence. In one orientation it will pass through, in another it will be rejected. This is similar to how sunglasses are able to eliminate glare by absorbing the polarized portion of the light.
DESCRIBING ELECTROMAGNETIC ENERGY
The terms light, electromagnetic waves, and radiation all refer to the same physical phenomenon: electromagnetic energy. This energy can be described by frequency, wavelength, or energy. All three are related mathematically such that if you know one, you can calculate the other two. Radio and microwaves are usually described in terms of frequency (Hertz), infrared and visible light in terms of wavelength (meters), and x-rays and gamma rays in terms of energy (electron volts). This is a scientific convention that allows the convenient use of units that have numbers that are neither too large nor too small.
The number of crests that pass a given point within one second is described as the frequency of the wave. One wave—or cycle—per second is called a Hertz (Hz), after Heinrich Hertz who established the existence of radio waves. A wave with two cycles that pass a point in one second has a frequency of 2 Hz.
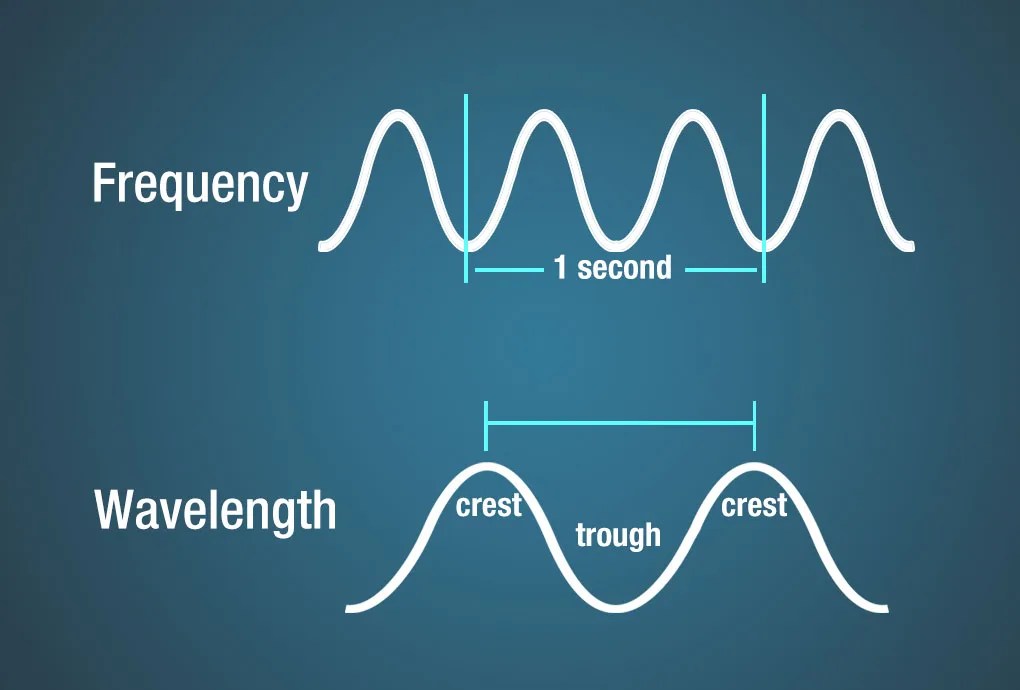
Electromagnetic waves have crests and troughs similar to those of ocean waves. The distance between crests is the wavelength. The shortest wavelengths are just fractions of the size of an atom, while the longest wavelengths scientists currently study can be larger than the diameter of our planet!
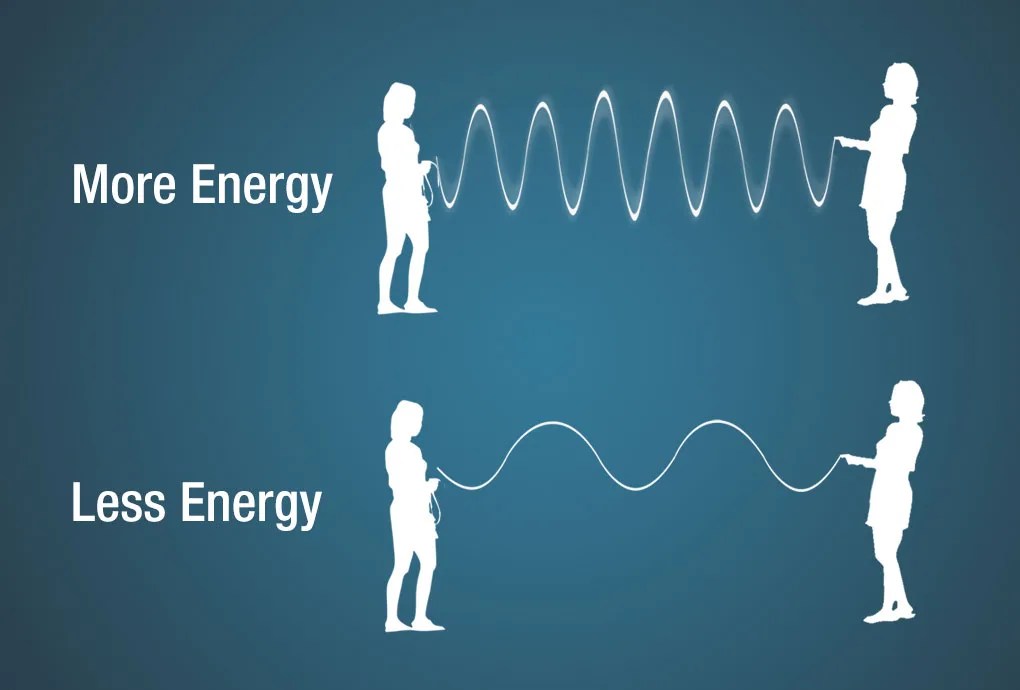
An electromagnetic wave can also be described in terms of its energy—in units of measure called electron volts (eV). An electron volt is the amount of kinetic energy needed to move an electron through one volt potential. Moving along the spectrum from long to short wavelengths, energy increases as the wavelength shortens. Consider a jump rope with its ends being pulled up and down. More energy is needed to make the rope have more waves.
Next: Wave Behaviors
National Aeronautics and Space Administration, Science Mission Directorate. (2010). Anatomy of an Electromagnetic Wave. Retrieved [insert date - e.g. August 10, 2016] , from NASA Science website: http://science.nasa.gov/ems/02_anatomy
Science Mission Directorate. "Anatomy of an Electromagnetic Wave" NASA Science . 2010. National Aeronautics and Space Administration. [insert date - e.g. 10 Aug. 2016] http://science.nasa.gov/ems/02_anatomy
Discover More Topics From NASA
James Webb Space Telescope
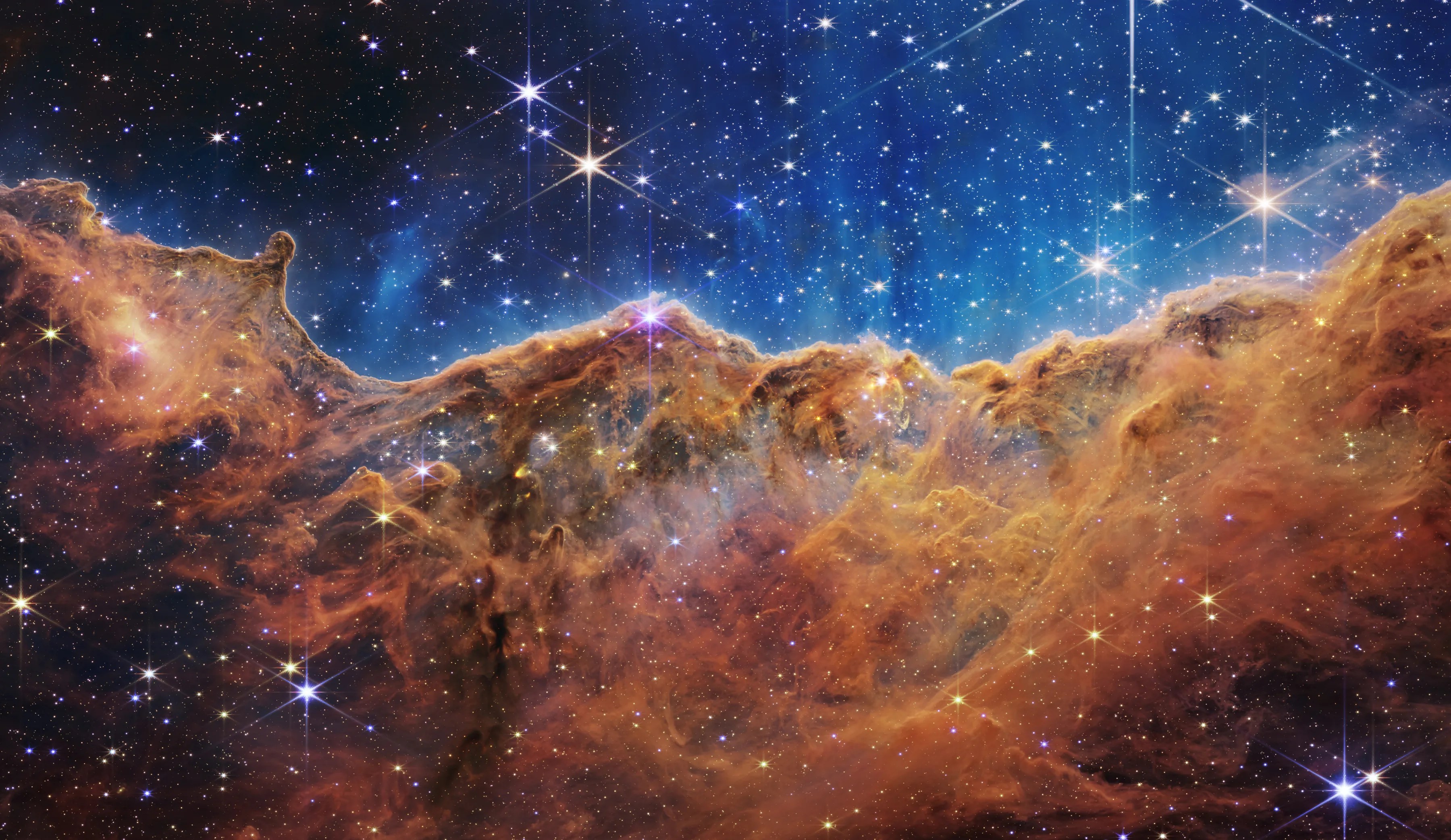
Perseverance Rover
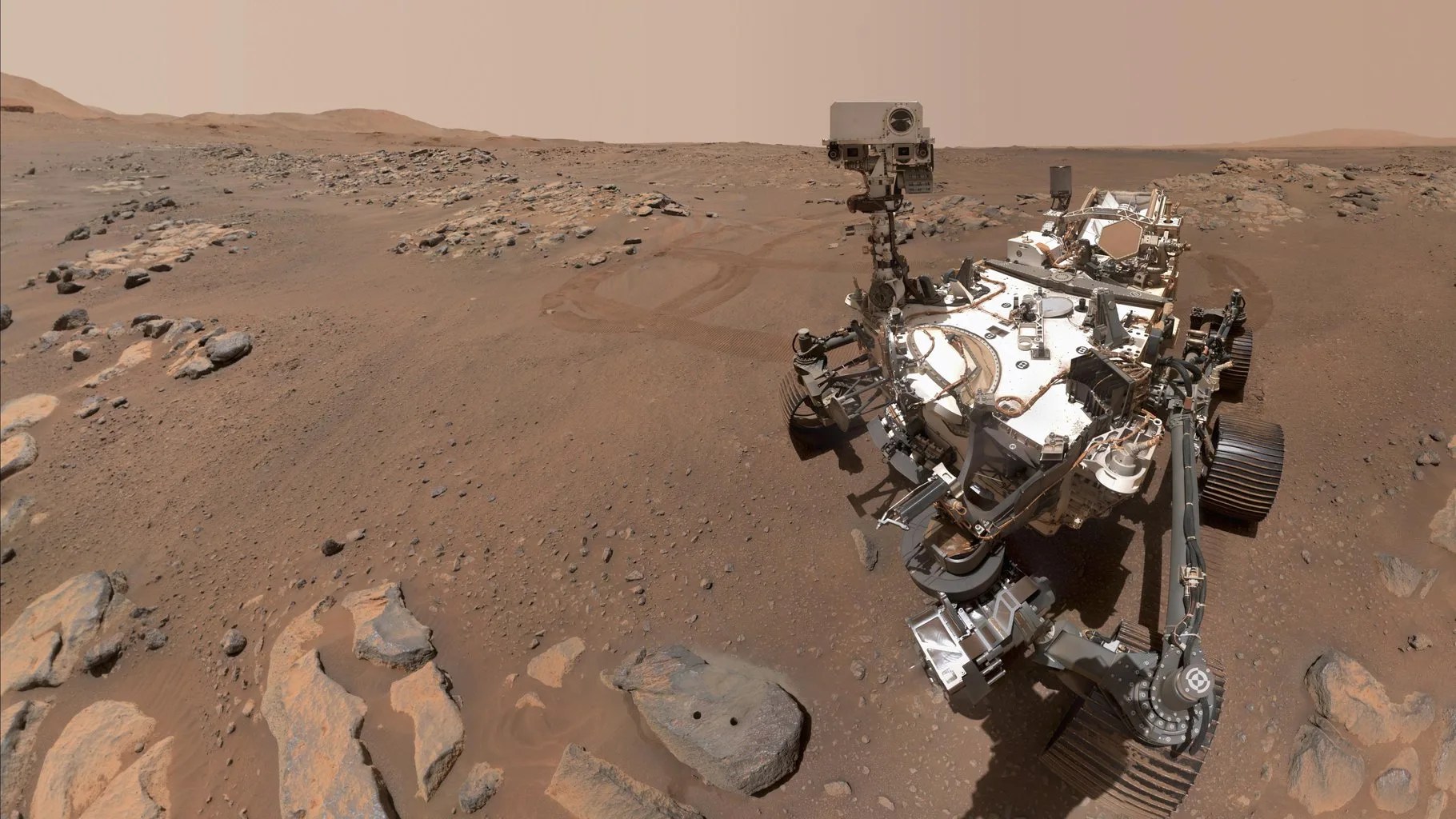
Parker Solar Probe
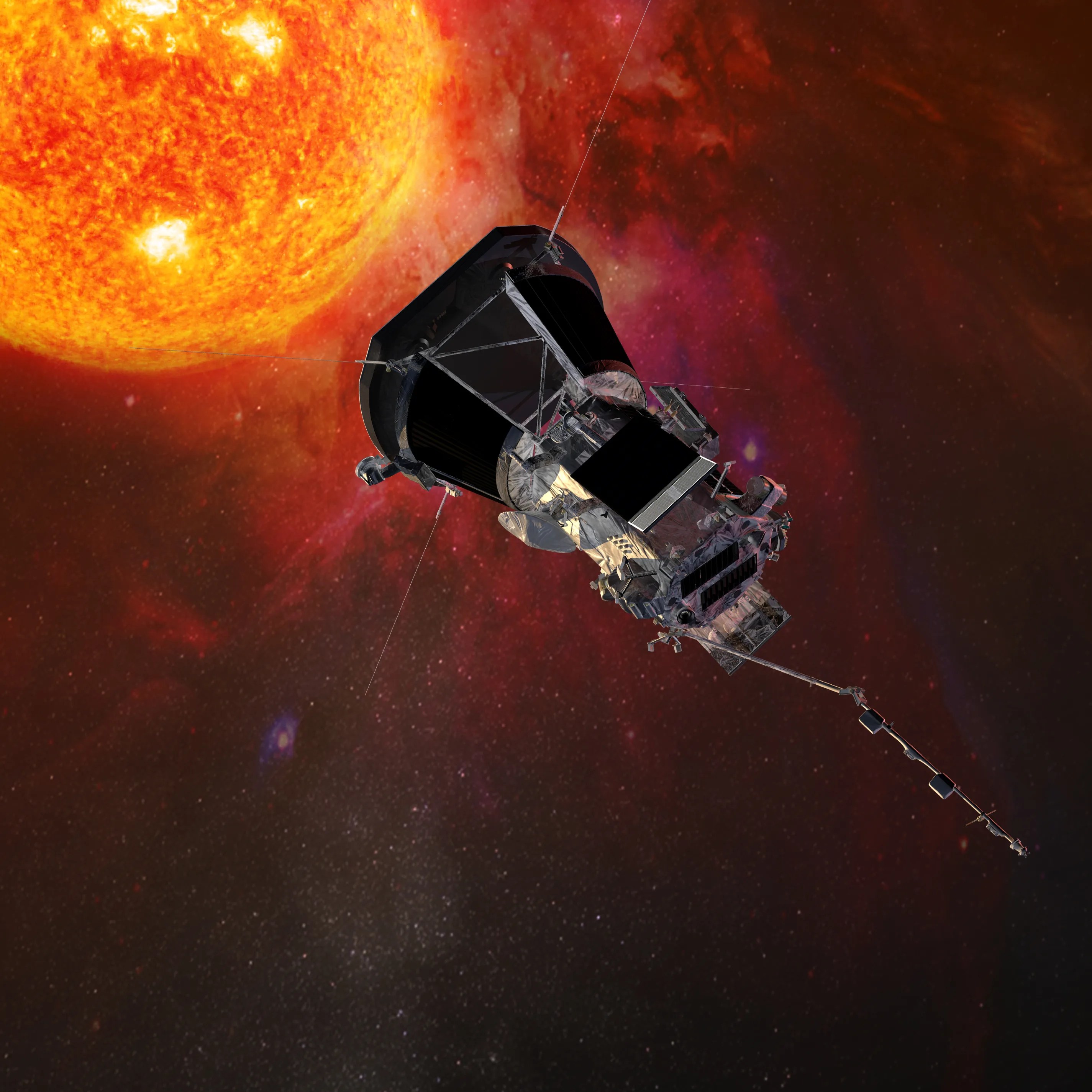
Electromagnetic Radiation
Claimed by Zoila de Leon (Fall 2023)
- 1.1 What is a Electromagnetic(EM) Radiation?
- 1.2 General Properties
- 1.3 Problem Solving Method and Equations
- 1.4 Fields Made by Charges and Fields Made by Monopoles
- 2 The EM Spectrum
- 3 Waves and Fields
- 4 A Mathematical Model
- 5 Connectedness: X-Rays
- 6.1 Practice Problems (new section by Zoila)
- 7 References
What is a Electromagnetic(EM) Radiation?
Electromagnetic radiation is a form of energy that is all around us and takes many forms, such as radio waves, microwaves, infrared, visible light, ultraviolet, x-rays, and gamma rays.
Before 1873, electricity and magnetism were thought to be two different forces. However, in 1873, Scottish Physicist James Maxwell developed his famous theory of electromagnetism. There are four main electro magnetic interactions according to Maxwell:
- The force of attraction or repulsion between electric charges is inversely proportional to the square of the distance between them
- Magnetic poles come in pairs that attract and repel each other much as electric charges do
- An electric current in a wire produces a magnetic field whose direction depends on the direction of the current
- A moving electric field produces a magnetic field, and vice versa
General Properties
The four Maxwell's Equations provide a complete description of possible spatial patterns of electric and magnetic field in space.
- The Ampere-Maxwell Law
- Gauss's Law
- Faraday's Law
Other than Maxwell's Four equations, there are general properties of all electromagnetic radiation:
- Electromagnetic radiation can travel through empty space. Most other types of waves must travel through some sort of substance. For example, sound waves need either a gas, solid, or liquid to pass through in order to be heard
- The speed of light is always a constant (3 x 10^8 m/s)
- Wavelengths are measured between the distances of either crests or troughs. It is usually characterized by the Greek symbol λ (lambda).
Electromagnetic waves are the self-propagating, mutual oscillation of electric and magnetic fields. The propagation of electromagnetic energy is often referred to as radiation. We can also say that the 'pulse' of these moving fields result in radiation (7).
The equation for propagation is E=cB with c being the speed of light. This equation is derived from combining the two equations E=vB and B=u0e0vE, proving that v is equal to 3e8 meters/second.
Problem Solving Method and Equations
To go about solving/analyzing mathematically an electromagnetic field using Maxwell's equations,this is how we proceed (7)
- Establish the space and time in which the electric and magnetic fields are present
- Check that Maxwell's equations can be applied in the situation above
- Check when the charge accelerates, it produces these fields and therefore radiation
- Show how these fields would interact with matter
The equation of the Radiative Electric Field is: E= 1/(4πe0)*-qa/(c^2r) where a is the acceleration of the particle, c is the speed of light and r is the distance from the original location of the charge to right before the kink. This kink happens on the electric field because of the slight delay when the charge is moved.
Fields Made by Charges and Fields Made by Monopoles
We can differentiate fields made by charges and the ones made by magnetic monopoles. (7) For fields made by charges, when the charge is
- at rest, E=1/r^2 and B=0
- constant speed, E=1/r^2 and B=1/r^2
- accelerating, E=1/r and B=1/r
For fields made by magnetic monopoles, the first point would have E and B switched.
The EM Spectrum
EM spectrum is a span of enormous range of wavelengths and frequencies. The EM spectrum is generally divided into 7 different regions, in order of decreasing wavelength and increasing energy and frequency. It ranges from Gamma rays to Long Radio Waves. Following are the lists of waves:
- Visible Light
- Infrared Rays
- Long radio waves
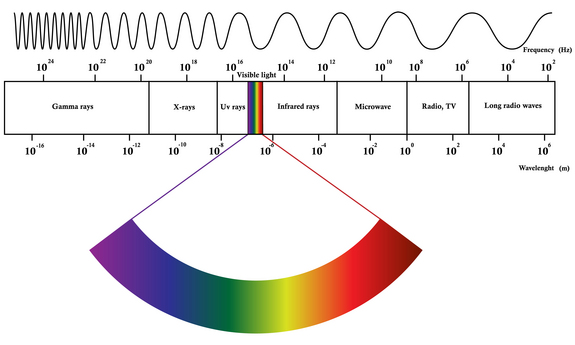
Although all these waves do different things, there is one thing in common : They all travel in waves.
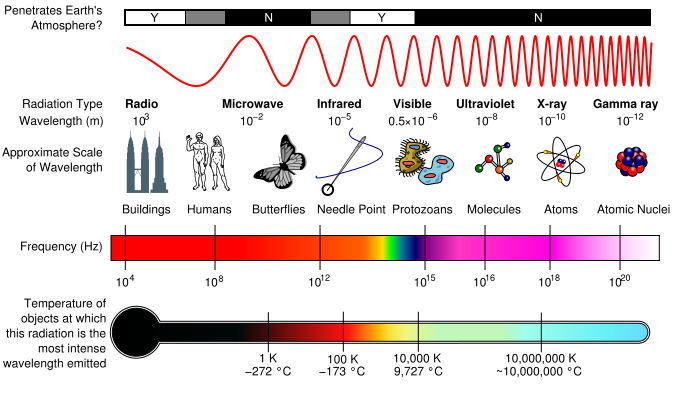
Infrared radiation can be released as heat or thermal energy. It can also be bounced back, which is called near infrared because of its similarities with visible light energy. Infrared Radiation is most commonly used in remote sensing as infrared sensors collect thermal energy, providing us with weather conditions.
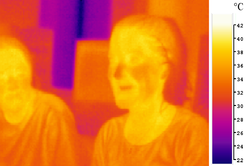
Visible Light is the only part of the electromagnetic spectrum that humans can see with a naked eye. This part of the spectrum includes a range of different colors that all represent a particular wavelength. Rainbows are formed in this way; light passes through matter in which it is absorbed or reflected based on its wavelength. As a result, some colors are reflected more than other, leading to the creation of a rainbow.
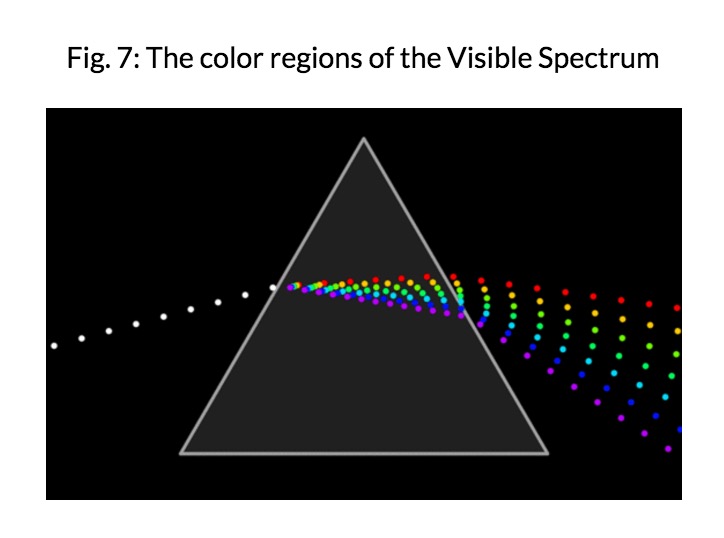
Waves and Fields
As we learned in class, electric field is produced when an electron is accelerating. Likewise, EM radiation is created when an atomic particle, like an electron, is accelerated by an electric field. The movement like this produces oscillating electric and magnetic fields, which travel at right angles to each other in a bundle of light energy called a photon. Photons travel in a harmonic wave at the fastest speed possible in the universe.
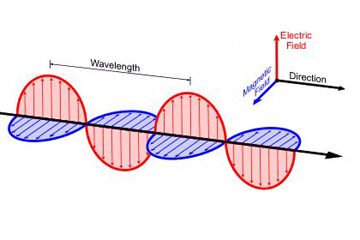
Electromagnetic waves are formed when an electric field couples with a magnetic field. Magnetic and electric fields of an electromagnetic wave are perpendicular to each other and to the direction of the wave.
A wavelength (in m) is the distance between two consecutive peaks of a wave. Frequency is the number of waves that form in a given length of time. A wavelength and frequency are interrelated. A short wavelength indicates that the frequency will be higher because one cycle can pass in a shorter amount of time. Likewise, a longer wavelength has a lower frequency because each cycle takes longer to complete.
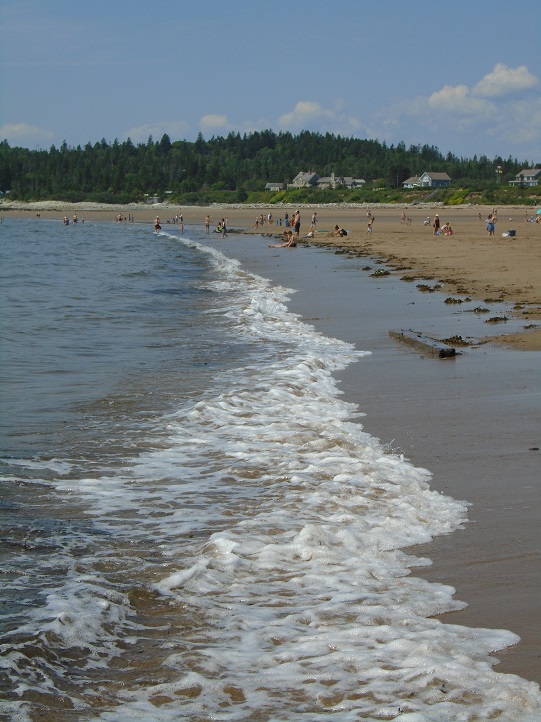
Waves can be classified according to their nature:
- Mechanical waves
- Electromagnetic waves
Mechanical Waves
Mechanical waves require a medium (matter) to travel through. Examples are sound waves, water waves, ripples in strings or springs.
Water Waves
Sound Waves
Electromagnetic Waves
Electromagnetic waves do not require a medium (matter) to travel through - they can travel through space. Examples are radio waves, visible light, x-rays.
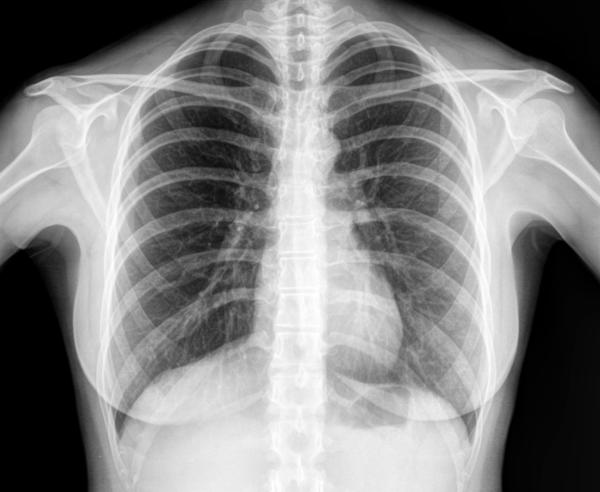
Radio Waves
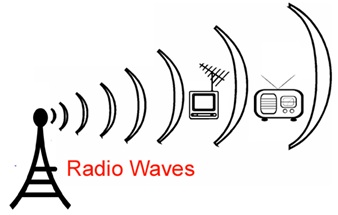
Visible Lights
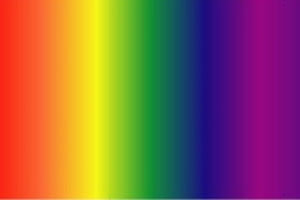
A Mathematical Model
The position of the particle is defined by a sine wave:
y = ymaxsin(wt)
Where w is the angular frequency
Amplitude is the distance from the maximum vertical displacement of the wave to the middle of the wave. The Amplitude of the sinusoidal Wave is the height of the peak in the wave measured from the zero line. This measures the magnitude of oscillation of a particular wave. The Amplitude is important because it tells you the intensity or brightness of a wave in comparison with other waves.
The period of the wave is the time between crests in seconds(s).
T = 2pi/w-----(units of seconds)
Frequency is the number of cycles per second, and is expressed as sec-1 or Hertz(Hz). Frequency is directly proportional to energy and can be express as "
E = hv where E is energy, h is Planck's constant ( 6.62607*10^-34J) and v is frequency
f = 1/T f = w/2pi----(Units Hertz)
Wavelength is the distance between crests in meters. Wavelength is equal to the speed of light times frequency. Longer wavelength waves such as radio waves carry low energy; this is why we can listen to the radio without any harmful consequences. Shorter wavelength waves such as x-rays carry higher energy that can be hazardous to our health.
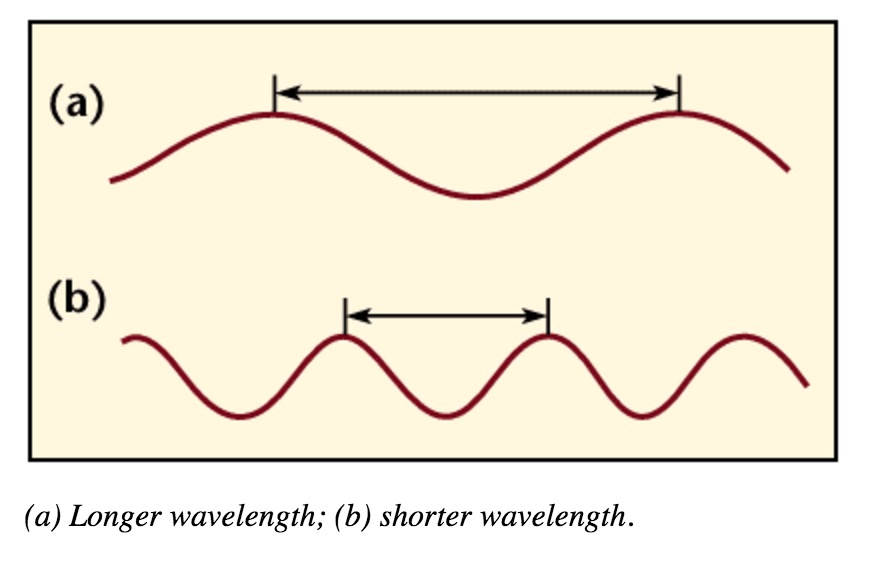
Wavelength and Frequency
The speed of light is the multiplication of the wavelength and frequency.
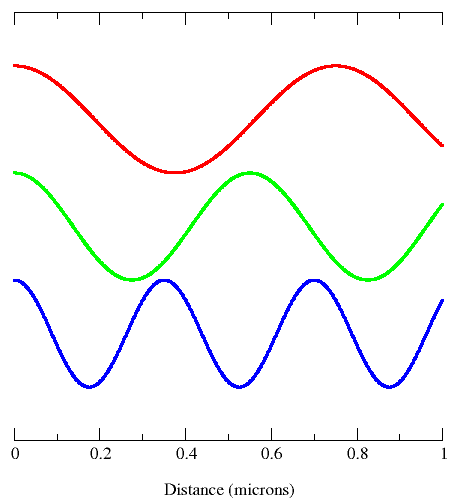
This diagram shows all properties of waves:
ENERGY FLUX
Is defined by the following equation:
Connectedness: X-Rays
Electromagnetic Radiation while commonly thought of as only including visible light, radio waves, UV waves, and gamma rays; also include X-rays. In 1895, X-rays were initially discovered by William Roentgen, who accidentally fell upon the most important discovery about his life (Figure 1). Roentgen was already working on cathode rays, and because of a fluorescent glow that occurred during his experiments, covered his experimental apparatus with heavy black paper. However, when he did this, he discovered a glow coming from a screen several feet away. Through many more experiments, he discovered that a new type of energy, not cathode rays, were the cause of the glow. He named them “x-rays” and received the 1901 Nobel Prize in Physics. Roentgen never patented his monumental discovery and as a result, numerous researchers set out to find a multitude of uses and capitalize on his work.
Primarily, people could now view objects that were hidden from plain view (i.e. scanners in airports). While X-rays are now used in 100’s of professions (security, chemistry, art galleries), its most important function is to view bones to determine abnormalities in humans. In fact, one of Roentgen’s first x-rays was of his wife’s hand (Figure 2). X-rays fall under the scope of electromagnetic radiation because, like all E.R. waves, it is comprised of photons. X-rays have wavelengths between 0.01 to 10 nanometers and fall between UV and Gamma Waves on the E.R. spectrum (Figure 3). There are two main methods in which an x-ray may be formed. Both require a vacuum-filled tube called an x-ray tube (Figure 4). With an anode on one end and a cathode on the other, an electric current is applied and a high energy electron is projected from the cathode, through the vacuum, and at the anode. In the characteristic x-ray generation approach, the electron from the cathode collides with an inner shell electron on an atom on the anode (Figure 5). Both of these electrons are ejected from the atom and an outer shell electron takes the place of the inner shell one. Because the outer electron must have a lower energy to fill the inner shell hole, it releases a photon with the equivalent energy of the difference between the two energy levels in the atom. This photon is the x-ray that is used to view objects such as bones.
In the Bremsstrahlung x-ray generation method, the electron from the cathode is slowed as it passes the nucleus of an atom at the anode (Figure 6). As it slows and its path is changed, the loses energy (kinetic energy). This energy is also released as a photon which is subsequently called an x-ray. Depending on the voltage and current of the tube and the material of the anode, different types (as in wavelengths and energy) of x-rays can be produced and each one. However, all X-rays will continue to pass through objects until it reaches a material dense that stops it. However, density of the material required depends on the energy of the x-ray. For example, during a medical x-ray, x-rays of a certain energy will pass through soft tissue (skin, organs, etc) but not through bones. The x-rays that pass through the soft tissue will strike the screen and the absence of the x-rays absorbed by the bones will cause a negative space on the screen. The areas where x-rays do not strike will form the image of the bone. While the principles remain the same, x-ray machines today use incredible sophisticated technology to specify the type of x-ray they want and have greatly increased in accuracy since Roentgen’s initial discovery.
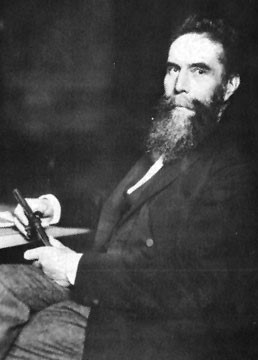
- Information and photographs are pulled from references 1 through 5 cited below*
Already, during the Ancient Greek and Roman times, light was studied as the presence of deflection and refraction were noticed. Electromagnetic radiation of wavelengths in the early 19th century. The discovery of infrared radiation is ascribed to astronomer William Herschel, who published his results in 1800 before the Royal Society of London. Herschel used a glass Triangular prism (optics)|prism to refract light from the Sun and detected invisible rays that caused heating beyond the red part of the spectrum, through an increase in the temperature recorded with a thermometer. These "calorific rays" were later termed infrared.
In 1801, Rohann Ritter, discovered the presence of ultraviolet light using salts. It was known that light could darken some silver halides and while doing so, he realized that the region beyond the violet bar (therefore ultraviolet) was more effective in changing the color of the halides. However,in 1864, while summarizing the theories of his time accumulating into his famous set of Maxwell equations, James Clerk Maxwell managed to deduce the speed of light being around 3e8 meters per second. This was instrumental in creating the rest of the spectrum.
In 1887-1888 Physicist Heinrich Hertz not only tried to measure the velocity and frequency of electromagnetic radiation waves at other parts of the known spectrum of the time, but he was also able to prove that Maxwell's findings were correct. He did this on the microwave radiation as well.
The discovery of X-rays occurred in 1895 by Wilhelm Rontgen when his barium platinocyanide detector screen began to glow under the presence of a discharge that passed through a cathode ray tube although the latter was completely covered. Once he determined its possible use, he tried to look at his wife's hand using this new discovery. However x-ray spectroscopy was not institutionalized until later by Karl Manne Siegbahn.
In 1900, Paul Villard discovered Gamma rays although he initially thought that they were particles similar to alpha and beta particles which were emitted during radiation. These 'particles' were later proven to be part of the electromagnetic spectrum.
Practice Problems (new section by Zoila)
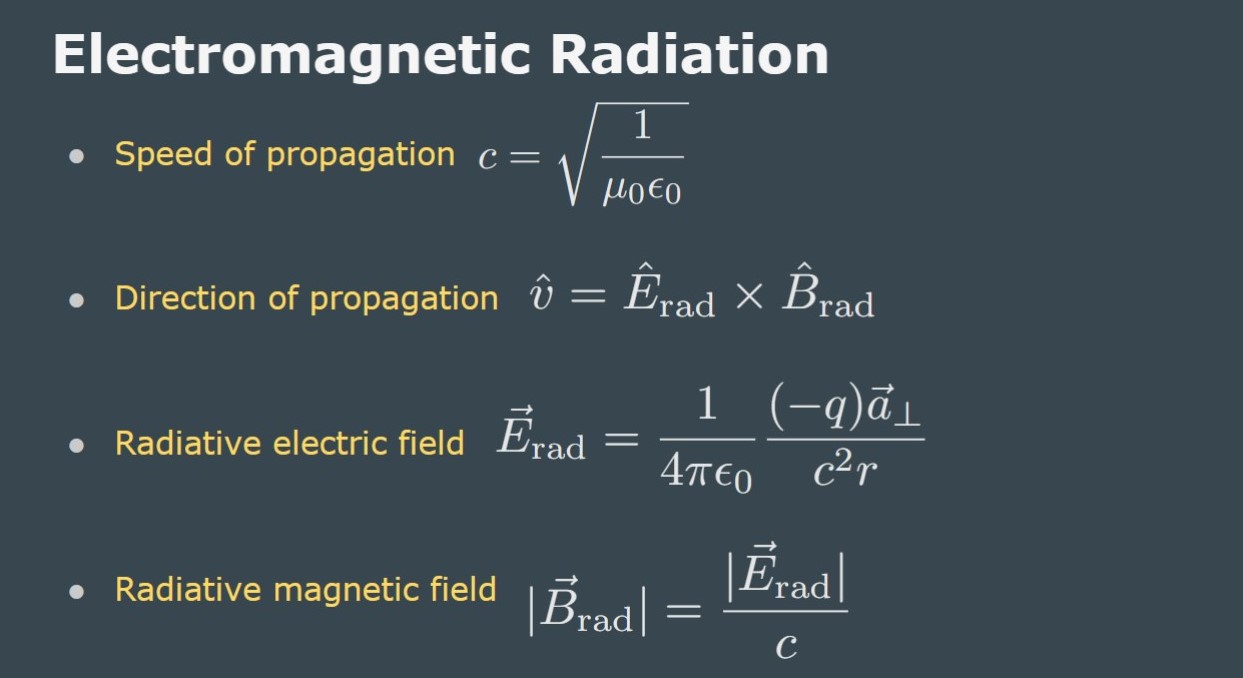
1. Elert, Glenn. "X-rays." X-rays – The Physics Hypertextbook. N.p., n.d. Web. 08 Apr. 2017. http://physics.info/x-ray/
2."X-rays." X-rays. N.p., n.d. Web. 08 Apr. 2017. http://www.physics.isu.edu/radinf/xray.htm
3. "Basics of X-ray PhysicsX-ray production." Welcome to Radiology Masterclass. N.p., n.d. Web. 08 Apr. 2017. http://www.radiologymasterclass.co.uk/tutorials/physics/x-ray_physics_production#top_2nd_img
4. "X-Rays." Image: Electromagnetic Spectrum. N.p., n.d. Web. 08 Apr. 2017. https://www.boundless.com/physics/textbooks/boundless-physics-textbook/electromagnetic-waves-23/the-electromagnetic-spectrum-165/x-rays-597-11175/images/electromagnetic-spectrum/
5. "This Month in Physics History." American Physical Society. N.p., n.d. Web. 08 Apr. 2017. https://www.aps.org/publications/apsnews/200111/history.cfm
6. Editors, Spectroscopy. “The Electromagnetic Spectrum: A History.” Spectroscopy Home, 27 Oct. 2017, www.spectroscopyonline.com/electromagnetic-spectrum-history?id=&sk=&date=&&pageID=4.
7. Chabay, Ruth W., and Bruce A. Sherwood. Matter & Interaction II: Electric & Magnetic Interactions, Version 1.2. John Wiley & Sons, 2003.
Navigation menu
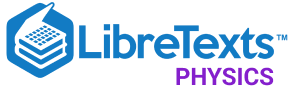
- school Campus Bookshelves
- menu_book Bookshelves
- perm_media Learning Objects
- login Login
- how_to_reg Request Instructor Account
- hub Instructor Commons
- Download Page (PDF)
- Download Full Book (PDF)
- Periodic Table
- Physics Constants
- Scientific Calculator
- Reference & Cite
- Tools expand_more
- Readability
selected template will load here
This action is not available.
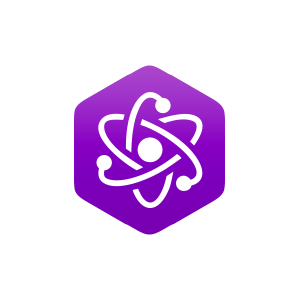
16.3: Plane Electromagnetic Waves
- Last updated
- Save as PDF
- Page ID 4450
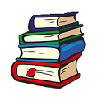
LEARNING OBJECTIVES
By the end of this section, you will be able to:
- Describe how Maxwell’s equations predict the relative directions of the electric fields and magnetic fields, and the direction of propagation of plane electromagnetic waves
- Explain how Maxwell’s equations predict that the speed of propagation of electromagnetic waves in free space is exactly the speed of light
- Calculate the relative magnitude of the electric and magnetic fields in an electromagnetic plane wave
- Describe how electromagnetic waves are produced and detected
Mechanical waves travel through a medium such as a string, water, or air. Perhaps the most significant prediction of Maxwell’s equations is the existence of combined electric and magnetic (or electromagnetic) fields that propagate through space as electromagnetic waves. Because Maxwell’s equations hold in free space, the predicted electromagnetic waves, unlike mechanical waves, do not require a medium for their propagation.
A general treatment of the physics of electromagnetic waves is beyond the scope of this textbook. We can, however, investigate the special case of an electromagnetic wave that propagates through free space along the x -axis of a given coordinate system.
Electromagnetic Waves in One Direction
An electromagnetic wave consists of an electric field, defined as usual in terms of the force per charge on a stationary charge, and a magnetic field, defined in terms of the force per charge on a moving charge. The electromagnetic field is assumed to be a function of only the x -coordinate and time. The y -component of the electric field is then written as \(E_y (x,t)\), the z -component of the magnetic field as \(B_z (x,t)\), etc. Because we are assuming free space, there are no free charges or currents, so we can set \(Q_{in} = 0\) and \(I = 0\) in Maxwell’s equations.
The transverse nature of electromagnetic waves
We examine first what Gauss’s law for electric fields implies about the relative directions of the electric field and the propagation direction in an electromagnetic wave. Assume the Gaussian surface to be the surface of a rectangular box whose cross-section is a square of side l and whose third side has length \(\Delta x\), as shown in Figure \(\PageIndex{1}\). Because the electric field is a function only of x and t , the y -component of the electric field is the same on both the top (labeled Side 2) and bottom (labeled Side 1) of the box, so that these two contributions to the flux cancel. The corresponding argument also holds for the net flux from the z -component of the electric field through Sides 3 and 4. Any net flux through the surface therefore comes entirely from the x -component of the electric field. Because the electric field has no y - or z -dependence, \(E_x(x,t)\) is constant over the face of the box with area A and has a possibly different value \(E_x (x + \Delta x, t)\) that is constant over the opposite face of the box.
Applying Gauss’s law gives
\[\text{Net flux} = - E_x (x,t) A + E_x (x + \Delta x, t) A = \dfrac{Q_{in}}{\epsilon_0} \label{16.13}\]
where \(A = l \times l\) is the area of the front and back faces of the rectangular surface. But the charge enclosed is \(Q_{in} = 0\), so this component’s net flux is also zero, and Equation \ref{16.13} implies \(E_x (x,t) = E_x (x + \Delta x, t)\) for any \(\Delta x\). Therefore, if there is an x -component of the electric field, it cannot vary with x . A uniform field of that kind would merely be superposed artificially on the traveling wave, for example, by having a pair of parallel-charged plates. Such a component \(E_x(x,t)\) would not be part of an electromagnetic wave propagating along the x -axis; so \(E_x(x,t) = 0\) for this wave. Therefore, the only nonzero components of the electric field are \(E_y(x,t)\) and \(E_z(x,t)\) perpendicular to the direction of propagation of the wave.
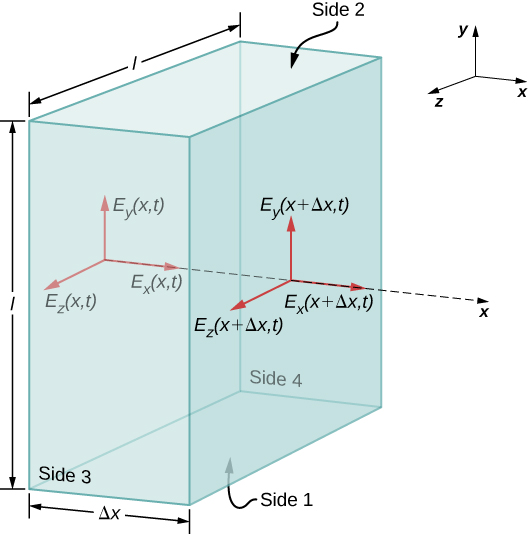
A similar argument holds by substituting E for B and using Gauss’s law for magnetism instead of Gauss’s law for electric fields. This shows that the B field is also perpendicular to the direction of propagation of the wave. The electromagnetic wave is therefore a transverse wave, with its oscillating electric and magnetic fields perpendicular to its direction of propagation.
The speed of propagation of electromagnetic waves
We can next apply Maxwell’s equations to the description given in connection with Figure 16.2.3 in the previous section to obtain an equation for the E field from the changing B field, and for the B field from a changing E field. We then combine the two equations to show how the changing E and B fields propagate through space at a speed precisely equal to the speed of light.
First, we apply Faraday’s law over Side 3 of the Gaussian surface, using the path shown in Figure \(\PageIndex{2}\). Because \(E_x(x,t) = 0\), we have
\[\oint \vec{E} \cdot d\vec{s} = - E_y(x,t)l + E_y(x + \Delta x,t)l.\]
Assuming \(\Delta x\) is small and approximating \(E_y (x + \Delta x,t)\) by
\[E_y (x + \Delta x,t) = E_y (x,t) + \dfrac{\partial E_y(x,t)}{\partial x} \Delta x,\]
\[\oint \vec{E} \cdot d\vec{s} = \dfrac{\partial E_y (x,t)}{\partial x} (l\Delta x).\]
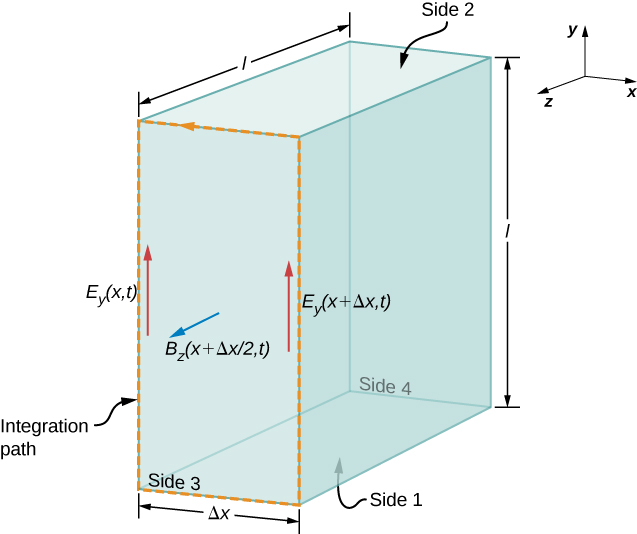
Because \(\Delta x\) is small, the magnetic flux through the face can be approximated by its value in the center of the area traversed, namely \(B_z\left(x + \dfrac{\Delta x}{2}, t\right)\). The flux of the B field through Face 3 is then the B field times the area,
\[\oint_S \vec{B} \cdot \vec{n} dA = B_z \left(x + \dfrac{\Delta x}{2}, t\right) (l \Delta x). \label{16.14}\]
From Faraday’s law ,
\[\oint \vec{E} \cdot d\vec{s} = -\dfrac{d}{dt} \int_S \vec{B} \cdot \vec{n} dA.\label{16.15}\]
Therefore, from Equations \ref{16.13} and \ref{16.14},
\[\dfrac{\partial E_y (x,t)}{\partial x} (l \Delta x) = - \dfrac{\partial}{\partial t} \left[ B_z \left( x + \dfrac{\Delta x}{2}, t\right) \right] (l\Delta x).\]
Canceling \(l \Delta x\) and taking the limit as \(\Delta x = 0\), we are left with
\[\dfrac{\partial E_y (x,t)}{\partial x} = - \dfrac{\partial B_z(x,t)}{\partial t}. \label{16.16}\]
We could have applied Faraday’s law instead to the top surface (numbered 2) in Figure \(\PageIndex{2}\), to obtain the resulting equation
\[\dfrac{\partial B_z(x,t)}{\partial t} = - \dfrac{\partial E_y (x,t)}{\partial x}. \label{16.17}\]
This is the equation describing the spatially dependent E field produced by the time-dependent B field.
Next we apply the Ampère-Maxwell law (with \(I = 0\)) over the same two faces (Surface 3 and then Surface 2) of the rectangular box of Figure \(\PageIndex{2}\). Applying Equation 16.2.16 ,
\[\oint \vec{B} \cdot d\vec{s} = \mu_0 \epsilon_0 (d/dt) \int_S \vec{E} \cdot n \, da\]
to Surface 3, and then to Surface 2, yields the two equations
\[\dfrac{\partial E_y (x,t)}{\partial x} = - \epsilon_0 \mu_0 \dfrac{\partial E_z (x,t)}{\partial t}, \label{16.18}\]
\[\dfrac{\partial B_z(x,t)}{\partial x} = - \epsilon_0 \mu_0 \dfrac{\partial E_y (x,t)}{\partial t}. \label{16.19}\]
These equations describe the spatially dependent B field produced by the time-dependent E field.
We next combine the equations showing the changing B field producing an E field with the equation showing the changing E field producing a B field. Taking the derivative of Equation \ref{16.16} with respect to x and using Equation \ref{16.26} gives
\[\dfrac{\partial^2E_y}{\partial x^2} = \dfrac{\partial}{\partial x}\left(\dfrac{\partial E_y}{\partial x}\right) = - \dfrac{\partial}{\partial x}\left(\dfrac{\partial B_z}{\partial t}\right) = - \dfrac{\partial}{\partial t}\left(\dfrac{\partial B_z}{\partial x}\right) = \dfrac{\partial}{\partial t}\left(\epsilon_0 \mu_0 \dfrac{\partial E_y}{\partial t}\right)\]
\[\dfrac{\partial^2E_y}{\partial x^2} = \epsilon_0 \mu_0 \dfrac{\partial^2 E_y}{\partial t^2}\]
This is the form taken by the general wave equation for our plane wave. Because the equations describe a wave traveling at some as-yet-unspecified speed c , we can assume the field components are each functions of x – ct for the wave traveling in the + x -direction, that is,
\[E_y (x,t) = f(\xi) \, where \, \xi = x - ct. \label{16.21}\]
It is left as a mathematical exercise to show, using the chain rule for differentiation, that Equations \ref{16.17} and \ref{16.18} imply
\[1 = \epsilon_0 \mu_0 c^2.\]
The speed of the electromagnetic wave in free space is therefore given in terms of the permeability and the permittivity of free space by
\[c = \dfrac{1}{\sqrt{\epsilon_0\mu_0}}. \label{16.22}\]
We could just as easily have assumed an electromagnetic wave with field components \(E_z (x,t)\) and \(B_y (x,t)\). The same type of analysis with Equation \ref{16.25} and \ref{16.24} would also show that the speed of an electromagnetic wave is \(c = 1/\sqrt{\epsilon_0\mu_0}\).
The physics of traveling electromagnetic fields was worked out by Maxwell in 1873. He showed in a more general way than our derivation that electromagnetic waves always travel in free space with a speed given by Equation \ref{16.18}. If we evaluate the speed \(c = \dfrac{1}{\sqrt{\epsilon_0\mu_0}}\), we find that
\[c = \dfrac{1}{\sqrt{\left(8.85 \times 10^{-12} \dfrac{C^2}{N \cdot m^2}\right)\left(4\pi \times 10^{-7} \dfrac{T \cdot m}{A}\right)}} = 3.00 \times 10^8 m/s,\]
which is the speed of light . Imagine the excitement that Maxwell must have felt when he discovered this equation! He had found a fundamental connection between two seemingly unrelated phenomena: electromagnetic fields and light.
Exercise \(\PageIndex{1}\)
The wave equation was obtained by (1) finding the E field produced by the changing B field, (2) finding the B field produced by the changing E field, and combining the two results. Which of Maxwell’s equations was the basis of step (1) and which of step (2)?
Faraday’s law
the Ampère-Maxwell law
How the E and B Fields Are Related
So far, we have seen that the rates of change of different components of the E and B fields are related, that the electromagnetic wave is transverse, and that the wave propagates at speed c . We next show what Maxwell’s equations imply about the ratio of the E and B field magnitudes and the relative directions of the E and B fields.
We now consider solutions to Equation \ref{16.16} in the form of plane waves for the electric field:
\[E_y(x,t) = E_0 \, \cos \, (kx - \omega t). \label{16.23}\]
We have arbitrarily taken the wave to be traveling in the +x -direction and chosen its phase so that the maximum field strength occurs at the origin at time \(t = 0\). We are justified in considering only sines and cosines in this way, and generalizing the results, because Fourier’s theorem implies we can express any wave, including even square step functions, as a superposition of sines and cosines.
At any one specific point in space, the E field oscillates sinusoidally at angular frequency \(\omega\) between \(+E_0\) and \(-E_0\) and similarly, the B field oscillates between \(+B_0\) and \(-B_0\). The amplitude of the wave is the maximum value of \(E_y(x,t)\). The period of oscillation T is the time required for a complete oscillation. The frequency f is the number of complete oscillations per unit of time, and is related to the angular frequency \(\omega\) by \(\omega = 2\pi f\). The wavelength \(\lambda\) is the distance covered by one complete cycle of the wave, and the wavenumber k is the number of wavelengths that fit into a distance of \(2\pi\) in the units being used. These quantities are related in the same way as for a mechanical wave:
\[\omega = 2\pi f, \, \, f = \dfrac{1}{T}, \, \, k = \dfrac{2\pi}{\lambda}, \, \, and \, \, c = f\lambda = \omega/k.\]
Given that the solution of \(E_y\) has the form shown in Equation \ref{16.20}, we need to determine the \(B\) field that accompanies it. From Equation \ref{16.24}, the magnetic field component \(B_z\) must obey
\[\dfrac{\partial B_z}{\partial t} = - \dfrac{\partial E_y}{\partial x}\]
\[\dfrac{\partial B_z}{\partial t} = - \dfrac{\partial}{\partial x} E_0 \, \cos \, (kx - \omega t) = kE_0 \, sin\, (kx - \omega t). \label{16.24}\]
Because the solution for the B -field pattern of the wave propagates in the + x -direction at the same speed c as the E- field pattern, it must be a function of \(k(x - ct) = kx - \omega t\). Thus, we conclude from Equation \ref{16.21} that \(B_z\) is
\[B_z(x,t) = \dfrac{k}{\omega} E_0 \, \cos \, (kx - \omega t) = \dfrac{1}{c}E_0 \, \cos \, (kx - \omega t).\]
These results may be written as
\[E_y(x,t) = E_0 \, \cos \, (kx - \omega t)\]
\[B_z(x,t) = B_0 \, \cos \, (kx - \omega t) \label{16.25}\]
\[\dfrac{E_y}{B_z} = \dfrac{E_0}{B_0} = c. \label{16.26}\]
Therefore, the peaks of the E and B fields coincide, as do the troughs of the wave, and at each point, the E and B fields are in the same ratio equal to the speed of light c . The plane wave has the form shown in Figure \(\PageIndex{3}\).
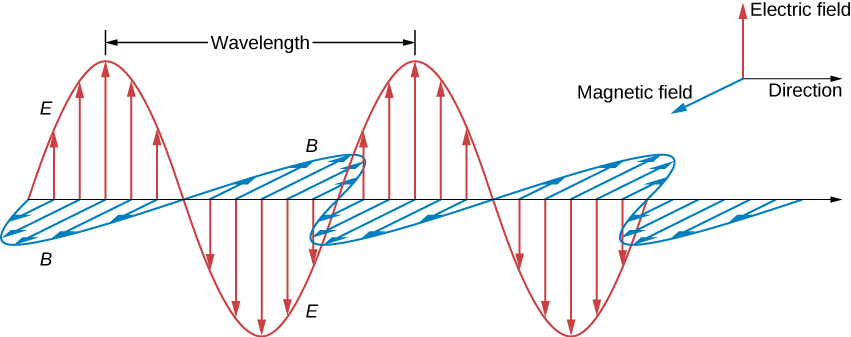
Example \(\PageIndex{1}\): Calculating B-Field Strength in an Electromagnetic Wave
What is the maximum strength of the B field in an electromagnetic wave that has a maximum E -field strength of 1000 V/m?
To find the B -field strength, we rearrange Equation \ref{16.23} to solve for \(B\), yielding
\[B = \dfrac{E}{c}. \nonumber\]
Solution We are given E , and c is the speed of light. Entering these into the expression for B yields
\[B = \dfrac{1000 \, V/m}{3.00 \times 10^8 \, m/s} = 3.33 \times 10^{-6} T. \nonumber\]
Significance
The B -field strength is less than a tenth of Earth’s admittedly weak magnetic field. This means that a relatively strong electric field of 1000 V/m is accompanied by a relatively weak magnetic field.
Changing electric fields create relatively weak magnetic fields. The combined electric and magnetic fields can be detected in electromagnetic waves, however, by taking advantage of the phenomenon of resonance, as Hertz did. A system with the same natural frequency as the electromagnetic wave can be made to oscillate. All radio and TV receivers use this principle to pick up and then amplify weak electromagnetic waves, while rejecting all others not at their resonant frequency.
Exercise \(\PageIndex{2}\)
What conclusions did our analysis of Maxwell’s equations lead to about these properties of a plane electromagnetic wave:
- the relative directions of wave propagation, of the E field, and of B field,
- the speed of travel of the wave and how the speed depends on frequency, and
- the relative magnitudes of the E and B fields.
The directions of wave propagation, of the E field, and of B field are all mutually perpendicular.
The speed of the electromagnetic wave is the speed of light \(c = 1/\sqrt{\epsilon_0\mu_0}\) independent of frequency.
The ratio of electric and magnetic field amplitudes is \(E/B = c\).
Production and Detection of Electromagnetic Waves
A steady electric current produces a magnetic field that is constant in time and which does not propagate as a wave. Accelerating charges, however, produce electromagnetic waves. An electric charge oscillating up and down, or an alternating current or flow of charge in a conductor, emit radiation at the frequencies of their oscillations. The electromagnetic field of a dipole antenna is shown in Figure \(\PageIndex{4}\). The positive and negative charges on the two conductors are made to reverse at the desired frequency by the output of a transmitter as the power source. The continually changing current accelerates charge in the antenna, and this results in an oscillating electric field a distance away from the antenna. The changing electric fields produce changing magnetic fields that in turn produce changing electric fields, which thereby propagate as electromagnetic waves. The frequency of this radiation is the same as the frequency of the ac source that is accelerating the electrons in the antenna. The two conducting elements of the dipole antenna are commonly straight wires. The total length of the two wires is typically about one-half of the desired wavelength (hence, the alternative name half-wave antenna ), because this allows standing waves to be set up and enhances the effectiveness of the radiation.
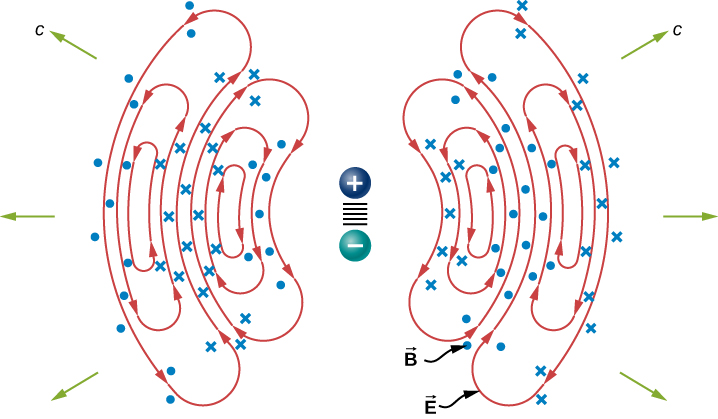
The electric field lines in one plane are shown. The magnetic field is perpendicular to this plane. This radiation field has cylindrical symmetry around the axis of the dipole. Field lines near the dipole are not shown. The pattern is not at all uniform in all directions. The strongest signal is in directions perpendicular to the axis of the antenna, which would be horizontal if the antenna is mounted vertically. There is zero intensity along the axis of the antenna. The fields detected far from the antenna are from the changing electric and magnetic fields inducing each other and traveling as electromagnetic waves. Far from the antenna, the wave fronts, or surfaces of equal phase for the electromagnetic wave, are almost spherical. Even farther from the antenna, the radiation propagates like electromagnetic plane waves.
The electromagnetic waves carry energy away from their source, similar to a sound wave carrying energy away from a standing wave on a guitar string. An antenna for receiving electromagnetic signals works in reverse. Incoming electromagnetic waves induce oscillating currents in the antenna, each at its own frequency. The radio receiver includes a tuner circuit, whose resonant frequency can be adjusted. The tuner responds strongly to tshe desired frequency but not others, allowing the user to tune to the desired broadcast. Electrical components amplify the signal formed by the moving electrons. The signal is then converted into an audio and/or video format.
Use this simulation to broadcast radio waves. Wiggle the transmitter electron manually or have it oscillate automatically. Display the field as a curve or vectors. The strip chart shows the electron positions at the transmitter and at the receiver.
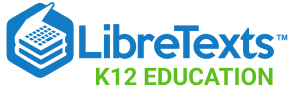
- school Campus Bookshelves
- menu_book Bookshelves
- perm_media Learning Objects
- login Login
- how_to_reg Request Instructor Account
- hub Instructor Commons
- Download Page (PDF)
- Download Full Book (PDF)
- Periodic Table
- Physics Constants
- Scientific Calculator
- Reference & Cite
- Tools expand_more
- Readability
selected template will load here
This action is not available.
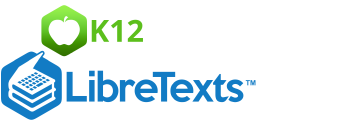
13.1: Electromagnetic Waves
- Last updated
- Save as PDF
- Page ID 2843
Did you ever wonder how a microwave works? It directs invisible waves of radiation toward the food placed inside of it. The radiation transfers energy to the food, causing it to get warmer. The radiation is in the form of microwaves, which are a type of electromagnetic waves.
What Are Electromagnetic Waves?
Electromagnetic waves are waves that consist of vibrating electric and magnetic fields. Like other waves, electromagnetic waves transfer energy from one place to another. The transfer of energy by electromagnetic waves is called electromagnetic radiation . Electromagnetic waves can transfer energy through matter or across empty space.
Q: How do microwaves transfer energy inside a microwave oven?
A: They transfer energy through the air inside the oven to the food.
May the Force Be With You
A familiar example may help you understand the vibrating electric and magnetic fields that make up electromagnetic waves. Consider a bar magnet, like the one in the Figure below. The magnet exerts magnetic force over an area all around it. This area is called a magnetic field. The field lines in the diagram represent the direction and location of the magnetic force. Because of the field surrounding a magnet, it can exert force on objects without touching them. They just have to be within its magnetic field.
Q: How could you demonstrate that a magnet can exert force on objects without touching them?
A: You could put small objects containing iron, such as paper clips, near a magnet and show that they move toward the magnet.
An electric field is similar to a magnetic field. It is an area of electrical force surrounding a positively or negatively charged particle. You can see electric fields in the following Figure below. Like a magnetic field, an electric field can exert force on objects over a distance without actually touching them.
How an Electromagnetic Wave Begins
An electromagnetic wave begins when an electrically charged particle vibrates. The Figure below shows how this happens. A vibrating charged particle causes the electric field surrounding it to vibrate as well. A vibrating electric field, in turn, creates a vibrating magnetic field. The two types of vibrating fields combine to create an electromagnetic wave.
How an Electromagnetic Wave Travels
As you can see in the Figure above, the electric and magnetic fields that make up an electromagnetic wave are perpendicular (at right angles) to each other. Both fields are also perpendicular to the direction that the wave travels. Therefore, an electromagnetic wave is a transverse wave. However, unlike a mechanical transverse wave, which can only travel through matter, an electromagnetic transverse wave can travel through empty space. When waves travel through matter, they lose some energy to the matter as they pass through it. But when waves travel through space, no energy is lost. Therefore, electromagnetic waves don’t get weaker as they travel. However, the energy is “diluted” as it travels farther from its source because it spreads out over an ever-larger area.
Electromagnetic Wave Interactions
When electromagnetic waves strike matter, they may interact with it in the same ways that mechanical waves interact with matter. Electromagnetic waves may:
- reflect, or bounce back from a surface;
- refract, or bend when entering a new medium;
- diffract, or spread out around obstacles.
Electromagnetic waves may also be absorbed by matter and converted to other forms of energy. Microwaves are a familiar example. When microwaves strike food in a microwave oven, they are absorbed and converted to thermal energy, which heats the food.
Sources of Electromagnetic Waves
The most important source of electromagnetic waves on Earth is the sun. Electromagnetic waves travel from the sun to Earth across space and provide virtually all the energy that supports life on our planet. Many other sources of electromagnetic waves depend on technology. Radio waves, microwaves, and X rays are examples. We use these electromagnetic waves for communications, cooking, medicine, and many other purposes.
Launch the Light Wave simulation below to help you visualize light as a transverse wave moving through the electromagnetic field. Be sure to adjust the wavelength band slider to observe waves of different sizes, such as Radio Waves and X-Rays. There is also an illustration of objects of comparable sizes next to the electromagnetic spectrum to help you imagine the sizes of these invisible light waves.
Interactive Element
- Electromagnetic waves are waves that consist of vibrating electric and magnetic fields. They transfer energy through matter or across space. The transfer of energy by electromagnetic waves is called electromagnetic radiation.
- The electric and magnetic fields of an electromagnetic wave are areas of electric or magnetic force. The fields can exert force over objects at a distance.
- An electromagnetic wave begins when an electrically charged particle vibrates. This causes a vibrating electric field, which in turn creates a vibrating magnetic field. The two vibrating fields together form an electromagnetic wave.
- An electromagnetic wave is a transverse wave that can travel across space as well as through matter. When it travels through space, it doesn’t lose energy to a medium as a mechanical wave does.
- When electromagnetic waves strike matter, they may be reflected, refracted, or diffracted. Or they may be absorbed by matter and converted to other forms of energy.
- The most important source of electromagnetic waves on Earth is the sun. Many other sources of electromagnetic waves depend on technology.
- What is an electromagnetic wave?
- Define electromagnetic radiation.
- Describe the electric and magnetic fields of an electromagnetic wave.
- How does an electromagnetic wave begin? How does it travel?
- Compare and contrast electromagnetic and mechanical transverse waves.
- List three sources of electromagnetic waves on Earth.
Explore More
Watch the electromagnetic wave animation and then answer the questions below.
- Identify the vibrating electric and magnetic fields of the wave.
- Describe the direction in which the wave is traveling.
Additional Resources
Study Guide: Wave Optics Study Guide
Real World Application: Printing with Light, The Incredible Hulk
PLIX: Play, Learn, Interact, eXplore: Energy Levels: Bohr's Atomic Model
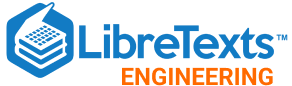
- school Campus Bookshelves
- menu_book Bookshelves
- perm_media Learning Objects
- login Login
- how_to_reg Request Instructor Account
- hub Instructor Commons
- Download Page (PDF)
- Download Full Book (PDF)
- Periodic Table
- Physics Constants
- Scientific Calculator
- Reference & Cite
- Tools expand_more
- Readability
selected template will load here
This action is not available.
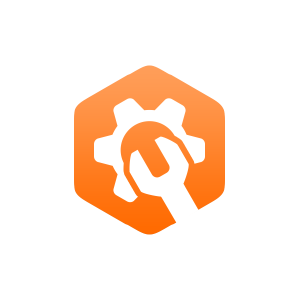
1.6: Electromagnetic Waves
- Last updated
- Save as PDF
- Page ID 18941

- Andrea M. Mitofsky
- Trine University
Maxwell's Equations
In this text, \(V\) and \(I\) denote DC voltage and current respectively while \(v\) and \(i\) denote AC or time varying voltage and current. In circuit analysis, we are unconcerned with what happens outside these wires. We are only interested in node voltages and currents through wires. Furthermore, the voltages and currents in the circuit are described as functions of time \(t\) but not position \((x, y, z)\). Devices like resistors, capacitors, and inductors too are assumed to be point-like and not extended with respect to position \((x, y, z)\). This set of assumptions is just a model. In reality, if two nodes in a circuit have a voltage difference between them, then necessarily a force is exerted on nearby charges not in the path of the circuit. This force per unit charge is the electric field intensity \(\overrightarrow{E}\). Similarly, if there is current flowing through a wire, there is necessarily a force exerted on electrons in nearby loops of wire, and this force per unit current element is the magnetic flux density \(\overrightarrow{B}\). Energy can be stored in an electric or magnetic field. In later chapters, we will discuss devices, including antennas, electro-optic devices, photovoltaic devices, lamps, and lasers, that convert energy of an electromagnetic field to or from electricity. Four interrelated vector quantities are used to describe electromagnetic fields. These vector fields are functions of position \((x, y, z)\) and time \(t\). The four vector fields are
- \(\overrightarrow{E}(x, y, z, t) =\) Electric field intensity in \(\frac{V}{m}\)
- \(\overrightarrow{D}(x, y, z, t) =\) Displacement field density in \(\frac{C}{m^2}\)
- \(\overrightarrow{H}(x, y, z, t) =\) Magnetic field intensity in \(\frac{A}{m}\)
- \(\overrightarrow{B}(x, y, z, t) =\) Magnetic flux density in \(\frac{Wb}{m^2}\)
In these expressions, \(V\) represents the units volts, \(C\) represents the units coulombs, \(A\) represents the units amperes, and \(Wb\) represents the units webers. Additional abbreviations for units are listed in Appendix B.
Coulomb's law:
\[\overrightarrow{F} = \frac{Q_1 Q_2 \hat a_r}{4 \pi \epsilon r^{2}} \nonumber \]
tells us that charged objects exert forces on other charged objects. In this expression, \(Q_1\) and \(Q_2\) are the magnitude of the charges in coulombs. The quantity \(\epsilon\) is the permittivity of the surrounding material in units farads per meter, and it is discussed further in 1.6.3 and 2.1.3. The quantity \(r\) is the distance between the charges in meters, and \(\hat a_r\) is a unit vector pointing along the direction between the charges. Force in newtons is represented by \(\overrightarrow{F}\). Opposite charges attract, and like charges repel. Electric field intensity is force per unit charge, so the electric field intensity due to a point charge is given by \[\overrightarrow{E} = \frac{Q \hat a_r}{4 \pi \epsilon r^{2}} \nonumber \]
These vector fields can describe forces on charges or currents in a circuit as well as outside the path of a circuit. Maxwell's equations relate time varying electric and magnetic fields. Maxwell's equations in differential form are:
\[\overrightarrow{\nabla} \times \overrightarrow{E} = -\frac{\partial \overrightarrow{B}}{\partial t} \quad \text{Faraday's Law} \nonumber \]
\[\overrightarrow{\nabla} \times \overrightarrow{H} = \overrightarrow{J} + \frac{\partial \overrightarrow{D}}{\partial t} \quad \text{Ampere's Law} \nonumber \]
\[\overrightarrow{\nabla} \cdot \overrightarrow{D} = \rho_{ch} \quad \text{Gauss's Law for the Electric Field} \nonumber \]
\[\overrightarrow{\nabla} \cdot \overrightarrow{B} = 0 \quad \text{Gauss's Law for the Magnetic Field} \nonumber \]
The additional quantities in Maxwell's equations are the volume current density \(\overrightarrow{J}\) in \(\frac{A}{m^2}\) and the charge density \(\rho_{ch}\) in \(\frac{C}{m^3}\). In this text, we will not be solving Maxwell's equations, but we will encounter references to them.
The quantity \(\overrightarrow{\nabla}\) is called the del operator. In Cartesian coordinates, it is given by
\[\overrightarrow{\nabla} =\hat a_x \frac{\partial}{\partial x} + \hat a_y \frac{\partial}{\partial y} + \hat a_z \frac{\partial}{\partial z}. \nonumber \]
When this operator acts on a scalar function, \(\overrightarrow{\nabla}f\), it is called the gradient. The gradient of a scalar function returns a vector representing the spatial derivative of the function, and it points in the direction of largest change in that function. In Maxwell's equations, \(\overrightarrow{\nabla}\) acts on vector, instead of scalar, functions. The operation \(\overrightarrow{\nabla} \times \overrightarrow{E}\) is called the curl, and the operation \(\overrightarrow{\nabla} \cdot \overrightarrow{E}\) is called the divergence. Both of these operations represent types of spatial derivatives of vector functions. The del operator obeys the identity
\[\nabla^2 =\overrightarrow{\nabla} \cdot \overrightarrow{\nabla}. \nonumber \]
The operation \(\nabla^2f\) is called the Laplacian of a scalar function, and it represents the spatial second derivative of that function.
Electromagnetic Waves in Free Space
Electromagnetic waves travel through empty space at the speed of light in free space, \(c = 2.998 \cdot 10^8 \frac{m}{s}\), and through other materials at speeds less than \(c\). For a sinusoidal electromagnetic wave, the speed of propagation is the product of the frequency and wavelength \[|\overrightarrow{v}| =f \lambda \nonumber \]
where \(|\overrightarrow{v}|\) is the magnitude of the velocity in \(\frac{m}{s}\), \(f\) is the frequency in Hz, and \(\lambda\) is the wavelength in meters. In free space, this becomes \[c =f \lambda. \nonumber \]
The speed of light in free space is related to two constants which describe free space. \[c = \frac{1}{\sqrt{\epsilon_0\mu_0}} \nonumber \]
The permittivity of free space is given by \(\epsilon_0 = 8.854 \cdot 10^{-12} \frac{F}{m}\) where \(F\) represents farads, and the permeability of free space is given by \(\mu_0 = 1.257 \cdot 10^{-6} \frac{H}{m}\) where \(H\) represents henries. (Constants specified in this section and in Appendix A are rounded to four significant digits.)
In free space, the electric field intensity \(\overrightarrow{E}\) and the displacement flux density \(\overrightarrow{D}\) are related by \(\epsilon_0\). \[\overrightarrow{D} = \mu_0 \overrightarrow{E} \nonumber \]
Relatedly in free space, the magnetic field intensity \(\overrightarrow{H}\) and the magnetic flux density \(\overrightarrow{B}\) are related by \(mu_0\). \[\overrightarrow{B} = \mu_0 \overrightarrow{H}. \nonumber \]
Electromagnetic Waves in Materials
Electromagnetic fields interact very differently with conductors and with insulators. Electromagnetic fields do not propagate into perfect conductors. Instead, charges and currents accumulate on the surface. While no materials are perfect conductors, commonly encountered metals like copper and aluminum are very good conductors. When these materials are placed in an external electromagnetic field, surface charges and currents build up, and the electromagnetic field in the material quickly approaches zero. Electromagnetic fields propagate through perfect insulators for long distances without decaying, and no charges or currents can accumulate on the surface because there are no electrons free from their atoms. In practical dielectrics, electromagnetic waves propagate long distances with very little attenuation. For example, optical electromagnetic waves remain strong enough to detect after propagating hundreds of kilometers through optical fibers made of pure silicon dioxide [10, p. 886].
Resistance \(R\) in ohms, capacitance \(C\) in farads, and inductance \(L\) in henries describe the electrical properties of devices. Resistivity \(\rho\) in \(\Omega m\), permittivity \(\epsilon\) in \(\frac {F}{m}\), and permeability \(\mu\) in \(\frac{H}{m}\) describe the electrical properties of materials. The quantities \(\rho\), \(\epsilon\), and \(\mu\) describe properties of materials alone while the quantities \(R\), \(C\), and \(L\) incorporate effects the material, shape, and size of a device.
Resistivity \(\rho\) is a measure of the inability of charges or electromagnetic waves to propagate through a material. Conductors have a very small resistivity while insulators have a large resistivity. Sometimes electrical conductivity, \(\sigma =\frac{1}{\rho}\) in units \(\frac{1}{\Omega m}\), is used in place of the resistivity. For a device made of a uniform material with length \(l\) and cross sectional area \(A\), resistance and resistivity are related by \[R =\frac{\rho l}{A}. \nonumber \]
Resistance is a measure of the inability of charges or electromagnetic waves to flow through a device while resistivity is a measure of the inability to flow through a material.
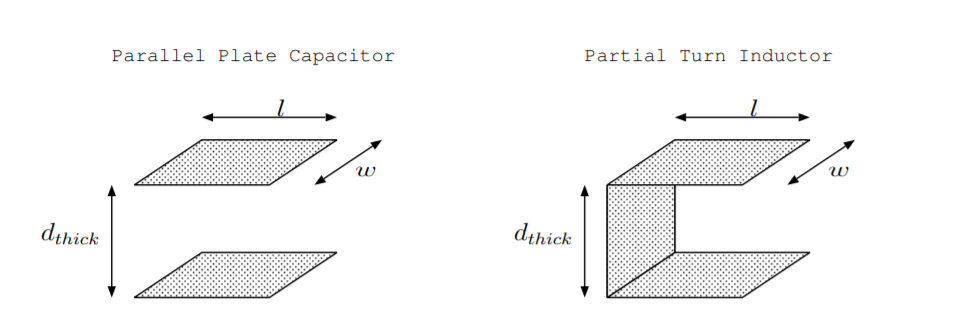
Permeability \(\mu\) is a measure of the ability of a material to store energy in the magnetic field due to currents distributed throughout the material. Materials can also be described by their relative permeability \(\mu_r\), a unitless measure. \[\mu_r = \frac{\mu}{\mu_0} \nonumber \]
While permeability describes a material, inductance describes a device. The magnetic flux density in a material is a scaled version of the magnetic field intensity.
\[\overrightarrow{B} =\mu\overrightarrow{H} \nonumber \]
Often insulators have permeabilities close to \(mu_0\) while conductors used to make permanent magnets have significantly larger permeabilities. The right part of Figure \(\PageIndex{1}\): shows a partial turn coil in a vacuum with length \(l\), thickness \(d_{thick}\), and width \(w\). The inductance and permeability of this device are related by [11, p. 311]
\[L =\frac{\mu d_{thick} l}{w}. \nonumber \]
Permittivity \(\epsilon\) is a measure of the ability of a material to store energy as an electric field due to charge separation distributed throughout the material. Materials can also be described by their relative permittivity \(\epsilon_r\), a unitless measure. \[\epsilon_r =\frac{\epsilon}{\epsilon_0} \nonumber \]
The displacement flux density in a material is a scaled version of the electric field intensity. \[\overrightarrow{D} =\epsilon\overrightarrow{E} \nonumber \]
Some insulators have a permittivity hundreds of times larger than the permittivity of free space. Permittivity is a measure of ability to store energy in a material while capacitance is a measure of the ability to store energy in in a device.
A uniform parallel plate capacitor with cross sectional area of plates \(A =l \cdot w\) and distance between plates \(d_{thick}\), is shown on the left part of Figure \(\PageIndex{1}\), and it has capacitance \[C =\frac{\epsilon A}{d_{thick}} \nonumber \] where \(\epsilon\) is the permittivity of the insulator between the plates.
Permittivity, permeability, and resistivity, depend on frequency. In some contexts, the frequency dependence can be ignored, and throughout most of this text, these quantities will be assumed to be constants. In other contexts, the frequency dependence can be quite significant. For example, the permittivity of semiconductor materials is a strong function of frequency for frequencies close to the semiconductor energy gap. The permittivity \(\epsilon(\omega)\) and resistivity \(\rho(\omega)\) are not independent. If one of them is known as a function of frequency and \(\mu\) is assumed constant, the other can be derived. This relationship is known as the Kramers Kronig relationship [10] or occasionally as the dielectric dispersion formula [15].
When discussing electrical properties of a device, resistance, inductance, and capacitance are combined into one complex measure, the impedance. Similarly, some authors find it convenient to combine resistivity, permittivity, and permeability into a pair of complex measures of the electrical properties of materials [6]. The complex permittivity is defined \(\epsilon^* =\epsilon+j\rho\), and the complex permeability is defined \(\mu^* =\mu+j\rho_{mag}\). The quantity \(\rho\) represents the resistivity which is a measure of the energy converted to heat as a charge flows through a material due to an applied electrical field. The quantity \(\rho_{mag}\) represents an analogous measure of energy converted to heat from currents in an applied magnetic field. Complex permittivity and permeability will not be used in this text.
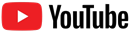
- TPC and eLearning
- Read Watch Interact
- What's NEW at TPC?
- Practice Review Test
- Teacher-Tools
- Subscription Selection
- Seat Calculator
- Ad Free Account
- Edit Profile Settings
- Classes (Version 2)
- Student Progress Edit
- Task Properties
- Export Student Progress
- Task, Activities, and Scores
- Metric Conversions Questions
- Metric System Questions
- Metric Estimation Questions
- Significant Digits Questions
- Proportional Reasoning
- Acceleration
- Distance-Displacement
- Dots and Graphs
- Graph That Motion
- Match That Graph
- Name That Motion
- Motion Diagrams
- Pos'n Time Graphs Numerical
- Pos'n Time Graphs Conceptual
- Up And Down - Questions
- Balanced vs. Unbalanced Forces
- Change of State
- Force and Motion
- Mass and Weight
- Match That Free-Body Diagram
- Net Force (and Acceleration) Ranking Tasks
- Newton's Second Law
- Normal Force Card Sort
- Recognizing Forces
- Air Resistance and Skydiving
- Solve It! with Newton's Second Law
- Which One Doesn't Belong?
- Component Addition Questions
- Head-to-Tail Vector Addition
- Projectile Mathematics
- Trajectory - Angle Launched Projectiles
- Trajectory - Horizontally Launched Projectiles
- Vector Addition
- Vector Direction
- Which One Doesn't Belong? Projectile Motion
- Forces in 2-Dimensions
- Being Impulsive About Momentum
- Explosions - Law Breakers
- Hit and Stick Collisions - Law Breakers
- Case Studies: Impulse and Force
- Impulse-Momentum Change Table
- Keeping Track of Momentum - Hit and Stick
- Keeping Track of Momentum - Hit and Bounce
- What's Up (and Down) with KE and PE?
- Energy Conservation Questions
- Energy Dissipation Questions
- Energy Ranking Tasks
- LOL Charts (a.k.a., Energy Bar Charts)
- Match That Bar Chart
- Words and Charts Questions
- Name That Energy
- Stepping Up with PE and KE Questions
- Case Studies - Circular Motion
- Circular Logic
- Forces and Free-Body Diagrams in Circular Motion
- Gravitational Field Strength
- Universal Gravitation
- Angular Position and Displacement
- Linear and Angular Velocity
- Angular Acceleration
- Rotational Inertia
- Balanced vs. Unbalanced Torques
- Getting a Handle on Torque
- Torque-ing About Rotation
- Properties of Matter
- Fluid Pressure
- Buoyant Force
- Sinking, Floating, and Hanging
- Pascal's Principle
- Flow Velocity
- Bernoulli's Principle
- Balloon Interactions
- Charge and Charging
- Charge Interactions
- Charging by Induction
- Conductors and Insulators
- Coulombs Law
- Electric Field
- Electric Field Intensity
- Polarization
- Case Studies: Electric Power
- Know Your Potential
- Light Bulb Anatomy
- I = ∆V/R Equations as a Guide to Thinking
- Parallel Circuits - ∆V = I•R Calculations
- Resistance Ranking Tasks
- Series Circuits - ∆V = I•R Calculations
- Series vs. Parallel Circuits
- Equivalent Resistance
- Period and Frequency of a Pendulum
- Pendulum Motion: Velocity and Force
- Energy of a Pendulum
- Period and Frequency of a Mass on a Spring
- Horizontal Springs: Velocity and Force
- Vertical Springs: Velocity and Force
- Energy of a Mass on a Spring
- Decibel Scale
- Frequency and Period
- Closed-End Air Columns
- Name That Harmonic: Strings
- Rocking the Boat
- Wave Basics
- Matching Pairs: Wave Characteristics
- Wave Interference
- Waves - Case Studies
- Color Addition and Subtraction
- Color Filters
- If This, Then That: Color Subtraction
- Light Intensity
- Color Pigments
- Converging Lenses
- Curved Mirror Images
- Law of Reflection
- Refraction and Lenses
- Total Internal Reflection
- Who Can See Who?
- Formulas and Atom Counting
- Atomic Models
- Bond Polarity
- Entropy Questions
- Cell Voltage Questions
- Heat of Formation Questions
- Reduction Potential Questions
- Oxidation States Questions
- Measuring the Quantity of Heat
- Hess's Law
- Oxidation-Reduction Questions
- Galvanic Cells Questions
- Thermal Stoichiometry
- Molecular Polarity
- Quantum Mechanics
- Balancing Chemical Equations
- Bronsted-Lowry Model of Acids and Bases
- Classification of Matter
- Collision Model of Reaction Rates
- Density Ranking Tasks
- Dissociation Reactions
- Complete Electron Configurations
- Elemental Measures
- Enthalpy Change Questions
- Equilibrium Concept
- Equilibrium Constant Expression
- Equilibrium Calculations - Questions
- Equilibrium ICE Table
- Ionic Bonding
- Lewis Electron Dot Structures
- Limiting Reactants
- Line Spectra Questions
- Mass Stoichiometry
- Measurement and Numbers
- Metals, Nonmetals, and Metalloids
- Metric Estimations
- Metric System
- Molarity Ranking Tasks
- Mole Conversions
- Name That Element
- Names to Formulas
- Names to Formulas 2
- Nuclear Decay
- Particles, Words, and Formulas
- Periodic Trends
- Precipitation Reactions and Net Ionic Equations
- Pressure Concepts
- Pressure-Temperature Gas Law
- Pressure-Volume Gas Law
- Chemical Reaction Types
- Significant Digits and Measurement
- States Of Matter Exercise
- Stoichiometry Law Breakers
- Stoichiometry - Math Relationships
- Subatomic Particles
- Spontaneity and Driving Forces
- Gibbs Free Energy
- Volume-Temperature Gas Law
- Acid-Base Properties
- Energy and Chemical Reactions
- Chemical and Physical Properties
- Valence Shell Electron Pair Repulsion Theory
- Writing Balanced Chemical Equations
- Mission CG1
- Mission CG10
- Mission CG2
- Mission CG3
- Mission CG4
- Mission CG5
- Mission CG6
- Mission CG7
- Mission CG8
- Mission CG9
- Mission EC1
- Mission EC10
- Mission EC11
- Mission EC12
- Mission EC2
- Mission EC3
- Mission EC4
- Mission EC5
- Mission EC6
- Mission EC7
- Mission EC8
- Mission EC9
- Mission RL1
- Mission RL2
- Mission RL3
- Mission RL4
- Mission RL5
- Mission RL6
- Mission KG7
- Mission RL8
- Mission KG9
- Mission RL10
- Mission RL11
- Mission RM1
- Mission RM2
- Mission RM3
- Mission RM4
- Mission RM5
- Mission RM6
- Mission RM8
- Mission RM10
- Mission LC1
- Mission RM11
- Mission LC2
- Mission LC3
- Mission LC4
- Mission LC5
- Mission LC6
- Mission LC8
- Mission SM1
- Mission SM2
- Mission SM3
- Mission SM4
- Mission SM5
- Mission SM6
- Mission SM8
- Mission SM10
- Mission KG10
- Mission SM11
- Mission KG2
- Mission KG3
- Mission KG4
- Mission KG5
- Mission KG6
- Mission KG8
- Mission KG11
- Mission F2D1
- Mission F2D2
- Mission F2D3
- Mission F2D4
- Mission F2D5
- Mission F2D6
- Mission KC1
- Mission KC2
- Mission KC3
- Mission KC4
- Mission KC5
- Mission KC6
- Mission KC7
- Mission KC8
- Mission AAA
- Mission SM9
- Mission LC7
- Mission LC9
- Mission NL1
- Mission NL2
- Mission NL3
- Mission NL4
- Mission NL5
- Mission NL6
- Mission NL7
- Mission NL8
- Mission NL9
- Mission NL10
- Mission NL11
- Mission NL12
- Mission MC1
- Mission MC10
- Mission MC2
- Mission MC3
- Mission MC4
- Mission MC5
- Mission MC6
- Mission MC7
- Mission MC8
- Mission MC9
- Mission RM7
- Mission RM9
- Mission RL7
- Mission RL9
- Mission SM7
- Mission SE1
- Mission SE10
- Mission SE11
- Mission SE12
- Mission SE2
- Mission SE3
- Mission SE4
- Mission SE5
- Mission SE6
- Mission SE7
- Mission SE8
- Mission SE9
- Mission VP1
- Mission VP10
- Mission VP2
- Mission VP3
- Mission VP4
- Mission VP5
- Mission VP6
- Mission VP7
- Mission VP8
- Mission VP9
- Mission WM1
- Mission WM2
- Mission WM3
- Mission WM4
- Mission WM5
- Mission WM6
- Mission WM7
- Mission WM8
- Mission WE1
- Mission WE10
- Mission WE2
- Mission WE3
- Mission WE4
- Mission WE5
- Mission WE6
- Mission WE7
- Mission WE8
- Mission WE9
- Vector Walk Interactive
- Name That Motion Interactive
- Kinematic Graphing 1 Concept Checker
- Kinematic Graphing 2 Concept Checker
- Graph That Motion Interactive
- Two Stage Rocket Interactive
- Rocket Sled Concept Checker
- Force Concept Checker
- Free-Body Diagrams Concept Checker
- Free-Body Diagrams The Sequel Concept Checker
- Skydiving Concept Checker
- Elevator Ride Concept Checker
- Vector Addition Concept Checker
- Vector Walk in Two Dimensions Interactive
- Name That Vector Interactive
- River Boat Simulator Concept Checker
- Projectile Simulator 2 Concept Checker
- Projectile Simulator 3 Concept Checker
- Hit the Target Interactive
- Turd the Target 1 Interactive
- Turd the Target 2 Interactive
- Balance It Interactive
- Go For The Gold Interactive
- Egg Drop Concept Checker
- Fish Catch Concept Checker
- Exploding Carts Concept Checker
- Collision Carts - Inelastic Collisions Concept Checker
- Its All Uphill Concept Checker
- Stopping Distance Concept Checker
- Chart That Motion Interactive
- Roller Coaster Model Concept Checker
- Uniform Circular Motion Concept Checker
- Horizontal Circle Simulation Concept Checker
- Vertical Circle Simulation Concept Checker
- Race Track Concept Checker
- Gravitational Fields Concept Checker
- Orbital Motion Concept Checker
- Angular Acceleration Concept Checker
- Balance Beam Concept Checker
- Torque Balancer Concept Checker
- Aluminum Can Polarization Concept Checker
- Charging Concept Checker
- Name That Charge Simulation
- Coulomb's Law Concept Checker
- Electric Field Lines Concept Checker
- Put the Charge in the Goal Concept Checker
- Circuit Builder Concept Checker (Series Circuits)
- Circuit Builder Concept Checker (Parallel Circuits)
- Circuit Builder Concept Checker (∆V-I-R)
- Circuit Builder Concept Checker (Voltage Drop)
- Equivalent Resistance Interactive
- Pendulum Motion Simulation Concept Checker
- Mass on a Spring Simulation Concept Checker
- Particle Wave Simulation Concept Checker
- Boundary Behavior Simulation Concept Checker
- Slinky Wave Simulator Concept Checker
- Simple Wave Simulator Concept Checker
- Wave Addition Simulation Concept Checker
- Standing Wave Maker Simulation Concept Checker
- Color Addition Concept Checker
- Painting With CMY Concept Checker
- Stage Lighting Concept Checker
- Filtering Away Concept Checker
- InterferencePatterns Concept Checker
- Young's Experiment Interactive
- Plane Mirror Images Interactive
- Who Can See Who Concept Checker
- Optics Bench (Mirrors) Concept Checker
- Name That Image (Mirrors) Interactive
- Refraction Concept Checker
- Total Internal Reflection Concept Checker
- Optics Bench (Lenses) Concept Checker
- Kinematics Preview
- Velocity Time Graphs Preview
- Moving Cart on an Inclined Plane Preview
- Stopping Distance Preview
- Cart, Bricks, and Bands Preview
- Fan Cart Study Preview
- Friction Preview
- Coffee Filter Lab Preview
- Friction, Speed, and Stopping Distance Preview
- Up and Down Preview
- Projectile Range Preview
- Ballistics Preview
- Juggling Preview
- Marshmallow Launcher Preview
- Air Bag Safety Preview
- Colliding Carts Preview
- Collisions Preview
- Engineering Safer Helmets Preview
- Push the Plow Preview
- Its All Uphill Preview
- Energy on an Incline Preview
- Modeling Roller Coasters Preview
- Hot Wheels Stopping Distance Preview
- Ball Bat Collision Preview
- Energy in Fields Preview
- Weightlessness Training Preview
- Roller Coaster Loops Preview
- Universal Gravitation Preview
- Keplers Laws Preview
- Kepler's Third Law Preview
- Charge Interactions Preview
- Sticky Tape Experiments Preview
- Wire Gauge Preview
- Voltage, Current, and Resistance Preview
- Light Bulb Resistance Preview
- Series and Parallel Circuits Preview
- Thermal Equilibrium Preview
- Linear Expansion Preview
- Heating Curves Preview
- Electricity and Magnetism - Part 1 Preview
- Electricity and Magnetism - Part 2 Preview
- Vibrating Mass on a Spring Preview
- Period of a Pendulum Preview
- Wave Speed Preview
- Slinky-Experiments Preview
- Standing Waves in a Rope Preview
- Sound as a Pressure Wave Preview
- DeciBel Scale Preview
- DeciBels, Phons, and Sones Preview
- Sound of Music Preview
- Shedding Light on Light Bulbs Preview
- Models of Light Preview
- Electromagnetic Radiation Preview
- Electromagnetic Spectrum Preview
- EM Wave Communication Preview
- Digitized Data Preview
- Light Intensity Preview
- Concave Mirrors Preview
- Object Image Relations Preview
- Snells Law Preview
- Reflection vs. Transmission Preview
- Magnification Lab Preview
- Reactivity Preview
- Ions and the Periodic Table Preview
- Periodic Trends Preview
- Intermolecular Forces Preview
- Melting Points and Boiling Points Preview
- Reaction Rates Preview
- Ammonia Factory Preview
- Stoichiometry Preview
- Gaining Teacher Access
- Tasks and Classes
- Tasks - Classic
- Subscription
- Subscription Locator
- 1-D Kinematics
- Newton's Laws
- Vectors - Motion and Forces in Two Dimensions
- Momentum and Its Conservation
- Work and Energy
- Circular Motion and Satellite Motion
- Thermal Physics
- Static Electricity
- Electric Circuits
- Vibrations and Waves
- Sound Waves and Music
- Light and Color
- Reflection and Mirrors
- About the Physics Interactives
- Task Tracker
- Usage Policy
- Newtons Laws
- Vectors and Projectiles
- Forces in 2D
- Momentum and Collisions
- Circular and Satellite Motion
- Balance and Rotation
- Electromagnetism
- Waves and Sound
- Forces in Two Dimensions
- Work, Energy, and Power
- Circular Motion and Gravitation
- Sound Waves
- 1-Dimensional Kinematics
- Circular, Satellite, and Rotational Motion
- Einstein's Theory of Special Relativity
- Waves, Sound and Light
- QuickTime Movies
- About the Concept Builders
- Pricing For Schools
- Directions for Version 2
- Measurement and Units
- Relationships and Graphs
- Rotation and Balance
- Vibrational Motion
- Reflection and Refraction
- Teacher Accounts
- Task Tracker Directions
- Kinematic Concepts
- Kinematic Graphing
- Wave Motion
- Sound and Music
- About CalcPad
- 1D Kinematics
- Vectors and Forces in 2D
- Simple Harmonic Motion
- Rotational Kinematics
- Rotation and Torque
- Rotational Dynamics
- Electric Fields, Potential, and Capacitance
- Transient RC Circuits
- Light Waves
- Units and Measurement
- Stoichiometry
- Molarity and Solutions
- Thermal Chemistry
- Acids and Bases
- Kinetics and Equilibrium
- Solution Equilibria
- Oxidation-Reduction
- Nuclear Chemistry
- NGSS Alignments
- 1D-Kinematics
- Projectiles
- Circular Motion
- Magnetism and Electromagnetism
- Graphing Practice
- About the ACT
- ACT Preparation
- For Teachers
- Other Resources
- Newton's Laws of Motion
- Work and Energy Packet
- Static Electricity Review
- Solutions Guide
- Solutions Guide Digital Download
- Motion in One Dimension
- Work, Energy and Power
- Frequently Asked Questions
- Purchasing the Download
- Purchasing the CD
- Purchasing the Digital Download
- About the NGSS Corner
- NGSS Search
- Force and Motion DCIs - High School
- Energy DCIs - High School
- Wave Applications DCIs - High School
- Force and Motion PEs - High School
- Energy PEs - High School
- Wave Applications PEs - High School
- Crosscutting Concepts
- The Practices
- Physics Topics
- NGSS Corner: Activity List
- NGSS Corner: Infographics
- About the Toolkits
- Position-Velocity-Acceleration
- Position-Time Graphs
- Velocity-Time Graphs
- Newton's First Law
- Newton's Second Law
- Newton's Third Law
- Terminal Velocity
- Projectile Motion
- Forces in 2 Dimensions
- Impulse and Momentum Change
- Momentum Conservation
- Work-Energy Fundamentals
- Work-Energy Relationship
- Roller Coaster Physics
- Satellite Motion
- Electric Fields
- Circuit Concepts
- Series Circuits
- Parallel Circuits
- Describing-Waves
- Wave Behavior Toolkit
- Standing Wave Patterns
- Resonating Air Columns
- Wave Model of Light
- Plane Mirrors
- Curved Mirrors
- Teacher Guide
- Using Lab Notebooks
- Current Electricity
- Light Waves and Color
- Reflection and Ray Model of Light
- Refraction and Ray Model of Light
- Classes (Legacy Version)
- Teacher Resources
- Subscriptions

- Newton's Laws
- Einstein's Theory of Special Relativity
- About Concept Checkers
- School Pricing
- Newton's Laws of Motion
- Newton's First Law
- Newton's Third Law
Propagation of an Electromagnetic Wave
Electromagnetic waves are waves which can travel through the vacuum of outer space. Mechanical waves, unlike electromagnetic waves, require the presence of a material medium in order to transport their energy from one location to another. Sound waves are examples of mechanical waves while light waves are examples of electromagnetic waves.
Electromagnetic waves are created by the vibration of an electric charge. This vibration creates a wave which has both an electric and a magnetic component. An electromagnetic wave transports its energy through a vacuum at a speed of 3.00 x 10 8 m/s (a speed value commonly represented by the symbol c ). The propagation of an electromagnetic wave through a material medium occurs at a net speed which is less than 3.00 x 10 8 m/s. This is depicted in the animation below.
The mechanism of energy transport through a medium involves the absorption and reemission of the wave energy by the atoms of the material. When an electromagnetic wave impinges upon the atoms of a material, the energy of that wave is absorbed. The absorption of energy causes the electrons within the atoms to undergo vibrations. After a short period of vibrational motion, the vibrating electrons create a new electromagnetic wave with the same frequency as the first electromagnetic wave. While these vibrations occur for only a very short time, they delay the motion of the wave through the medium. Once the energy of the electromagnetic wave is reemitted by an atom, it travels through a small region of space between atoms. Once it reaches the next atom, the electromagnetic wave is absorbed, transformed into electron vibrations and then reemitted as an electromagnetic wave. While the electromagnetic wave will travel at a speed of c (3 x 10 8 m/s) through the vacuum of interatomic space, the absorption and reemission process causes the net speed of the electromagnetic wave to be less than c. This is observed in the animation below.
The actual speed of an electromagnetic wave through a material medium is dependent upon the optical density of that medium. Different materials cause a different amount of delay due to the absorption and reemission process. Furthermore, different materials have their atoms more closely packed and thus the amount of distance between atoms is less. These two factors are dependent upon the nature of the material through which the electromagnetic wave is traveling. As a result, the speed of an electromagnetic wave is dependent upon the material through which it is traveling.
For more information on physical descriptions of waves, visit The Physics Classroom Tutorial . Detailed information is available there on the following topics:
Mechanical vs. Electromagnetic Waves Wavelike Behaviors of Light The EM and Visible Spectra Light Absorption, Reflection, and Transmission Optical Density and Light Speed
Return to List of Animations
Electromagnetic Waves
Electromagnetic waves were first postulated by James Clerk Maxwell and subsequently confirmed by Heinrich Hertz. Electromagnetic waves are created as a result of vibrations between an electric and a magnetic field. In this article, we will explore the definition and formation of electromagnetic waves along with the graphical and mathematical representations of electromagnetic waves in detail.
What Are Electromagnetic Waves?
Electromagnetic waves are also known as EM waves. Electromagnetic radiations are composed of electromagnetic waves that are produced when an electric field comes in contact with the magnetic field. It can also be said that electromagnetic waves are the composition of oscillating electric and magnetic fields. Electromagnetic waves are solutions of Maxwell’s equations, which are the fundamental equations of electrodynamics.
Read More: Maxwell’s Equations
How Are Electromagnetic Waves Formed?
- Generally, an electric field is produced by a charged particle. A force is exerted by this electric field on other charged particles. Positive charges accelerate in the direction of the field and negative charges accelerate in a direction opposite to the direction of the field.
- The Magnetic field is produced by a moving charged particle. A force is exerted by this magnetic field on other moving particles. The force on these charges is always perpendicular to the direction of their velocity and therefore only changes the direction of the velocity, not the speed.
- So, the electromagnetic field is produced by an accelerating charged particle. Electromagnetic waves are nothing but electric and magnetic fields travelling through free space with the speed of light c. An accelerating charged particle is when the charged particle oscillates about an equilibrium position. If the frequency of oscillation of the charged particle is f, then it produces an electromagnetic wave with frequency f. The wavelength λ of this wave is given by λ = c/f. Electromagnetic waves transfer energy through space.
Graphical Representation of Electromagnetic Waves
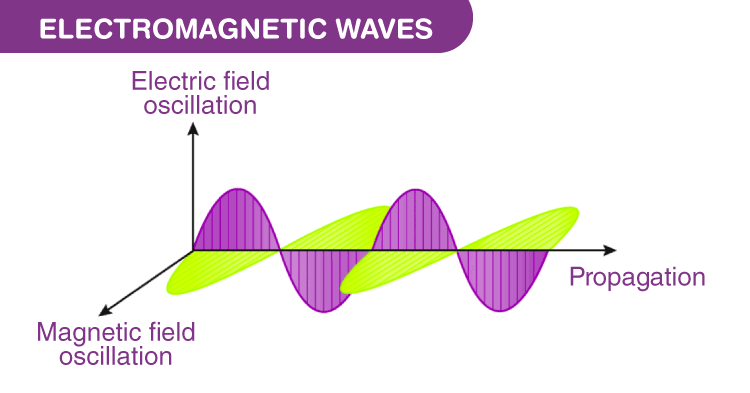
Mathematical Representation of Electromagnetic Wave
A plane Electromagnetic wave travelling in the x-direction is of the form
\(\begin{array}{l}E(x,t)=E_{max}\cos (kx-\omega t+\Phi )\end{array} \)
\(\begin{array}{l}B(x,t)=B_{max}\cos (kx-\omega t+\Phi )\end{array} \)
In the electromagnetic wave, E is the electric field vector and B is the magnetic field vector.
Maxwell gave the basic idea of Electromagnetic radiations, while Hertz experimentally confirmed the existence of an electromagnetic wave.
The direction of propagation of the electromagnetic wave is given by the vector cross product of the electric field and magnetic field. It is given as:
\(\begin{array}{l}\vec{E}\times \vec{B}\end{array} \) .
Read More: Magnetic Field
Electromagnetic Wave Equation
- The electromagnetic wave equation describes the propagation of electromagnetic waves in a vacuum or through a medium.
- The electromagnetic wave equation is a second-order partial differential equation.
- It is a 3D form of the wave equation.
- The homogeneous form of the equation is written as
\(\begin{array}{l}(\upsilon ^{2}_{ph}\bigtriangledown^{2}-\frac{\partial^2 }{\partial t^2})E=0\end{array} \) \(\begin{array}{l}(\upsilon ^{2}_{ph}\bigtriangledown^{2}-\frac{\partial^2 }{\partial t^2})B=0\end{array} \)
Intensity of an Electromagnetic Wave
\(\begin{array}{l}I=\frac{P}{A}=\frac{1}{2}c\epsilon _{0}E_{0}^{2}\\ \\ =\frac{1}{2}\frac{c}{\mu _{0}}B_{0}^{2}\end{array} \)
Speed of Electromagnetic Waves in Free Space
It is given by \(\begin{array}{l}C=\frac{1}{\sqrt{(\mu _{0}\epsilon _{0})}}\end{array} \)
\(\begin{array}{l}\mu _{0}\end{array} \) is called absolute permeability. Its value is \(\begin{array}{l}1.257\times10^{-6}TmA^{-1}\end{array} \)
\(\begin{array}{l}\epsilon _{0}\end{array} \) is called absolute permittivity. Its value is \(\begin{array}{l}8.854\times 10^{-12}C^{2}N^{-1}m^{-2}\end{array} \)
C is the velocity of light in vacuum = velocity of electromagnetic waves in free space = \(\begin{array}{l}3\times 10^{8}ms^{-1}\end{array} \)
Electromagnetic Spectrum
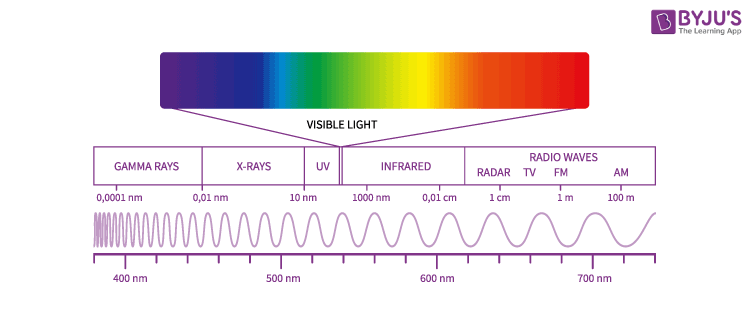
Electromagnetic waves are classified according to their frequency f or according to their wavelength \(\begin{array}{l}\lambda =\frac{c}{f}.\end{array} \)
The wavelength ranges of different lights are as follows,
For visible light – approx. 400 nm to approx. 700 nm
For violet light – approx. 400 nm
For red light – approx. 700 nm
For more insights, stay tuned with BYJU ’s and fall in love with learning.
Applications of Electromagnetic Waves
Following are a few applications of electromagnetic waves:
- Electromagnetic radiations can transmit energy in a vacuum or using no medium at all.
- Electromagnetic waves play an important role in communication technology.
- Electromagnetic waves are used in RADARS.
- UV rays are used to detect forged bank notes. Real banknotes don’t turn fluorescent under UV light.
- Infrared radiation is used for night vision and is used in security cameras.
Frequently Asked Questions
Name the property of an electromagnetic wave which is dependent on the medium in which it is travelling..
Velocity of an electromagnetic wave is a property which is dependent on the medium in which it is travelling. Other properties such as frequency, time period, and wavelength are dependent on the source that is producing the wave.
What is the wavelength of the photon of infrared light with the frequency 2.5 x 10 14 Hz?
Since infrared light is a part of electromagnetic spectrum, the relation between the wavelength, frequency, and velocity is given by the formula: \(\begin{array}{l}c=f\lambda\end{array} \) Consider c = 3 x 10 8 m.s -1 . Substituting the values, we get wavelength = 1.2 x 10 -6 m.
State if the given statement is true or false: Radio waves and X-rays both are on the electromagnetic spectrum and can travel at the same speed.
The given statement is true. Any wave from the electromagnetic spectrum travels at a constant speed of light. Other properties such as frequency, energy, and wavelength vary depending on the type of wave and the source producing them. But the velocity remains constant.
What is the reason behind photons travelling at a speed of light while the other particles cannot?
Photons can travel at a speed of light while other particles cannot because they do not have mass.
State if the given statement is true or false: High frequency propagation is used so as to increase the accuracy.
The given statement is true. It is true that for increasing the accuracy, the frequency of propagation needs to be high.
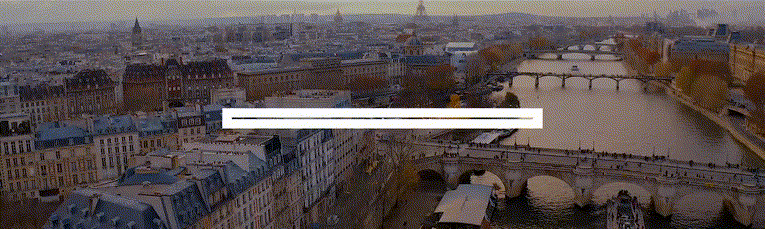
What is the sequence for the propagation of electromagnetic waves?
The sequence for the propagation of electromagnetic waves is the generation, propagation, reflection, and reception.
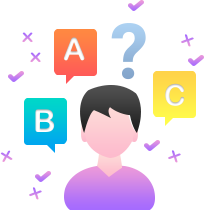
Put your understanding of this concept to test by answering a few MCQs. Click ‘Start Quiz’ to begin!
Select the correct answer and click on the “Finish” button Check your score and answers at the end of the quiz
Visit BYJU’S for all Physics related queries and study materials
Your result is as below
Request OTP on Voice Call
Leave a Comment Cancel reply
Your Mobile number and Email id will not be published. Required fields are marked *
Post My Comment

Very much helpful content is available on this site of Byjus
exellent good help in study easy concept

- Share Share
Register with BYJU'S & Download Free PDFs
Register with byju's & watch live videos.


An official website of the United States government
Official websites use .gov A .gov website belongs to an official government organization in the United States.
- JetStream home
- Layers of the Atmosphere
- Air Pressure
- The Transfer of Heat Energy
- Energy Balance
- Hydrologic Cycle
- Precipitation
- Layers of the Ocean
- Ocean Circulations
- The Sea Breeze
- The Marine Layer
- Rip Current Safety
- Global Atmospheric Circulations
- The Jet Stream
- Climate vs. Weather
- Climate Zones
- How clouds form
- The Core Four
- The Basic Ten
- Cloud Chart
- Color of Clouds
- Parcel Theory
- Stability-Instability
- Radiosondes
- Skew-T Log-P Diagrams
- Skew-T Plots
- Skew-T Examples
- Pressure vs. Elevation
- Longwaves and Shortwaves
- Basic Wave Patterns
- Common Features
- Thickness Charts
- Weather Models
- Surface Weather Plots
- Norwegian Cyclone Model
- Types of Weather Phenomena
Electromagnetic waves
- The Atmospheric Window
- Weather Satellites
- How radar works
- Volume Coverage Patterns (VCP)
- Radar Images: Reflectivity
- Radar Images: Velocity
- Radar Images: Precipitation
- Inter-Tropical Convergence Zone
- Classification
- NHC Forecasts
- Hazards & Safety
- Damage Potential
- ENSO Pacific Impacts
- ENSO Weather Impacts
- Ingredients for a Thunderstorm
- Life Cycle of a Thunderstorm
- Types of Thunderstorms
- Hazard: Hail
- Hazard: Damaging wind
- Hazard: Tornadoes
- Hazard: Flash Floods
- Staying Ahead of the Storms
- How Lightning is Created
- The Positive and Negative Side of Lightning
- The Sound of Thunder
- Lightning Safety
- Frequently Asked Questions
- Types of Derechos
- Where and When
- Notable Derechos
- Keeping Yourself Safe
- Historical Context
- Causes: Earthquakes
- Causes: Landslides
- Causes: Volcanoes
- Causes: Weather
- Propagation
- Detection, Warning, and Forecasting
- Preparedness and Mitigation: Communities
- Preparedness and Mitigation: Individuals (You!)
- Select NOAA Tsunami Resources
- Weather Forecast Offices
- River Forecast Centers
- Center Weather Service Units
- Regional Offices
- National Centers for Environmental Prediction
- NOAA Weather Radio
- NWS Careers: Educational Requirements
- Weather Glossary
- Weather Acronyms
- Learning Lessons
- Electromagnetic Waves
- Atmospheric Absorption
Electromagnetic waves are a form of radiation that travel though the universe. They are formed when an electric field (Fig. 1 red arrows) couples with a magnetic field (Fig.1 blue arrows).
Both electricity and magnetism can be static (respectively, what holds a balloon to the wall or a refrigerator magnet to metal), but when they change or move together, they make waves. Magnetic and electric fields of an electromagnetic wave are perpendicular to each other and to the direction of the wave.
Unlike sound waves, which must travel through matter by bumping molecules into each other like dominoes (and thus can not travel through a vacuum like space), electromagnetic waves do not need molecules to travel. They can travel through air, solid objects, and even space, making them very useful for a lot of technologies.
When you listen to the radio, connect to a wireless network, or cook dinner in a microwave oven, you are using electromagnetic waves. Radio waves and microwaves are two types of electromagnetic waves. They only differ from each other in wavelength – the distance between one wave crest to the next.
While most of this energy is invisible to us, we can see the range of wavelengths that we call light. This visible part of the electromagnetic spectrum consists of the colors that we see in a rainbow – red, orange, yellow, green, blue, indigo, and violet. Each of these colors also corresponds to a different measurable wavelength of light.
Waves in the electromagnetic spectrum vary in size from very long radio waves that are the length of buildings to very short gamma-rays that are smaller than the nucleus of an atom.
Their size is related to their energy. The smaller the wavelength, the higher the energy. For example, a brick wall blocks the relatively larger and lower-energy wavelengths of visible light but not the smaller, more energetic x-rays. A denser material such as lead, however, can block x-rays.
While it’s commonly said that waves are "blocked" by certain materials, the correct understanding is that wavelengths of energy are absorbed by the material. This understanding is critical to interpreting data from weather satellites because the atmosphere also absorbs some wavelengths while allowing others to pass through.
How does astronomy use the electromagnetic spectrum?
There is more to light than meets the eye, and it teaches us a lot about the universe.
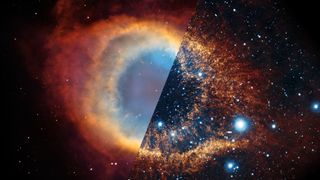
- Radio waves
- Submillimeter waves
- Ultraviolet
Additional resources
Bibliography.
Parts of the electromagnetic spectrum invisible to human eyes reveal a vast amount of information about the universe, but it took a long time for astronomers to learn how to view it.
For thousands of years, humans were looking up at the star-studded night sky using just their eyes sensitive to the optical wavelength of the electromagnetic spectrum . The first telescopes, invented in the early 17th century, enhanced the ability of human eyes by magnifying distant objects.
But as physicists started discovering in the 19th century that there are other, invisible, types of light in the natural world around us, astronomers realized that there must be such light emanating also from the universe .
Related: Astronomy: The oldest scientific discipline
Today, astronomers know that the majority of radiation, or light, present in the universe is invisible to human eyes. By looking at the universe in all possible wavelengths, scientists are piecing together a complex picture of the unfathomably vast cosmic environment that we are a part of. It took, however, decades, for instruments to be developed that could detect this invisible radiation from celestial sources.
Here we explain what different parts of the electromagnetic spectrum teach us about the universe.
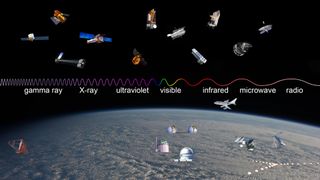
What do radio waves teach us about the universe?
Radio astronomy studies cosmic radiation with the longest wavelengths (from less than 0.4 inches to several miles, or 1 centimeter to several kilometers) and was the first kind of astronomy developed that relies on wavelengths other than optical light.
The discovery that radio waves from bodies in the universe lash our planet was made completely by accident. In 1933, a young American radio engineer Karl Jansky, an employee of the famous telephone company Bell Laboratories, was tasked to search for sources of unexplained hiss that sometimes interfered with transmissions of radio messages across the Atlantic Ocean. Jansky found that while some of this noise was coming from sources on Earth , such as nearby thunderstorms, there was a type of signal, constantly picked up by his experimental antennas, that appeared to be coming from what we know today is the center of our Milky Way galaxy, the region where the black hole Sagittarius A* resides. Systematic exploration of the radio universe began soon thereafter.
Astronomers have discovered since that radio waves are emitted by spinning electrons and emanate from all sorts of environments that have the ability to make those electrons spin, Affelia Wibisono, an astronomer at the Royal Observatory Greenwich in the U.K., told Space.com.
"Typically, when you detect radio waves, you're looking at electrons moving through a magnetic field," Wibisono said. "But ionized gas can emit radio waves as well."
By tracing the structure of radio wave-emitting clouds, astronomers were able to map out the entire structure of our galaxy, the Milky Way, as well as other nearby galaxies . They could determine areas with high concentrations of hot young stars , but also study objects obscured by dust, such as black holes that hide in galactic centers. Highly magnetized bodies, such as fast-spinning stellar remnants called pulsars are prime targets for radio astronomy as they send out powerful flashes of radio waves as they spin like superfast cosmic lighthouses.
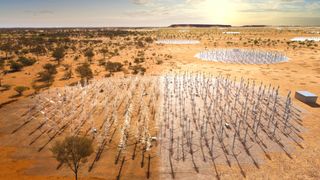
Famous radio telescopes
As radio waves are the type of electromagnetic radiation with the longest wavelengths, radio telescopes have to be rather large. Vast arrays of radio-antennas, such as the Karl G. Jansky Very Large Array in New Mexico that consists of 28 dishes each 82-foot-wide (25 meters), are the technological standard today. By combining multiple antennas, astronomers create telescopes that have immense apertures that equal the distance between the array's most distant parts, thus enabling the scientist to detect the faintest signals with the best possible resolution.
The Square Kilometer Array (SKA), currently constructed across two locations in Australia and South Africa, will be the world's largest radio telescope by a significant margin once it comes online around 2028. With its thousands of dishes and dipole antennas spanning thousands of square miles of remote land, SKA will survey large areas of the sky at once and detect the faintest signals coming from the farthest reaches of the universe.
The Event Horizon Telescope , famous for taking photographs of black holes , is also a radio telescope, or rather a worldwide network of radio telescopes with an aperture equalling the size of our planet.
Unlike some other types of wavelengths, radio waves mostly penetrate Earth's atmosphere with ease, allowing astronomers to base their equipment on the planet's surface.
However, due to the ubiquity of radio communication technologies in the modern world, radio telescopes are at risk of getting confused by human-made signals. SKA, for example, will therefore be surrounded by a radio-quiet zone where no cell phones and no radio equipment will be allowed.
Constantly searching for better ways to study the universe, astronomers are now seriously considering building a radio telescope on the far side of the moon . Removed from Earth-based sources of human-made radio noise, as well as from Earth's ionosphere (the upper part of the atmosphere which contains ionized gas that absorbs and distorts some cosmic radio signals), such an observatory would provide scientists with the deepest and most undisturbed views into the earliest epoch of the universe.
What do microwaves teach us about the universe?
The next electromagnetic spectrum band after radio waves are microwaves. As microwaves cover wavelengths between 3.3 feet and 0.04 inches (1 meter and 1 millimeter), the first discoveries of cosmic microwaves were actually made by radio telescopes.
Microwaves have a special, although rather limited place in astronomy. According to the European Space Agency (ESA), the whole sky glows uniformly in microwaves with what has been identified as the cosmic microwave background .
This uniformness is unseen in other wavelengths, which reveal the sky in dots and regions of varying brightness. In fact, cosmic microwave radiation is so odd that the researchers who first discovered it in the 1960s (completely by accident during experiments with echo balloons) originally thought it was produced by a telescope defect.
Subsequent research, however, confirmed that the microwave hum was coming from space and that it was nothing less than the residue of radiation released by the Big Bang , the enormous explosion which created the universe some 13.8 billion years ago.
This radiation was originally released in the form of highly energetic, short-wavelength X-rays, but since it took so long to reach us, the so-called redshift effect caused by the expansion of the universe has stretched this wavelength all the way into microwaves.
Microwaves reveal the universe as it looked in its earliest stages. The most sensitive surveys were able to go as far as distinguishing the denser regions of gas and dust that subsequently produced the first galaxies.
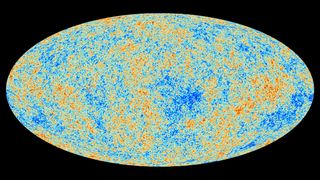
Famous microwave telescopes
Microwaves get mostly absorbed by Earth's atmosphere, which means they are best studied by space-based telescopes.
In 1989, after the initial crude Earth-based detections of cosmic microwaves, NASA sent the first dedicated microwave-observing satellite — the Cosmic Background Explorer (COBE) — into space. COBE measured differences in the temperature of the microwave background in various regions. COBE's successor, the Wilkinson Microwave Anisotropy Probe (WMAP), launched in 2003, further improved the level of detail of this cosmic microwave map. These observations helped to determine the universe's age with greater precision, according to the European Space Agency (ESA) , and define the amounts of different types of matter that the universe contained in its earliest years.
ESA's Planck mission , launched in 2009, then completed the task of creating the most accurate map of the cosmic microwave background, which, ESA said, is to some extent "definitive," as some of the measurements cannot be further improved.
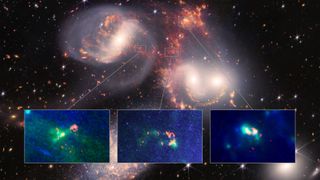
What do submillimeter waves teach us about the universe?
The submillimeter wavelength sits between the millimeter and infrared ranges. As the name suggests, submillimeter waves have lengths shorter than 1 mm, or 0.04 inches, and up to a few hundred micrometers. Observations in this range partially overlap with the longest wavelengths of the infrared spectrum.
The use of submillimeter wavelengths in astronomy is relatively recent, according to Astronomy Cast . Detectors used to detect submillimeter radiation are quite similar to those used in radio astronomy, but thousands of times smaller. Technology therefore had to progress enough to make these detectors possible.
Speaking to the Astronomy Cast, an astronomy podcast, American astronomer Pamela Gay said that the use of submillimeter waves in astronomy is limited to certain types of objects and phenomena.
Submillimeter waves penetrate through clouds of molecular gas and dust into star-forming regions, which are obscured from the view of of optical telescopes.
In submillimeter waves, astronomers can observe universe's "natural lasers," regions where highly charged electrons emit laser light as they discharge some of their energy, said Gay. These natural lasers, sometimes called masers , are usually observed in a special type of pulsating variable stars called the Mira stars .
Submillimeter waves are also good at pointing astronomers to some interesting types of organic molecules and do a good job analyzing cold objects such as comets in the solar system , said Gay.
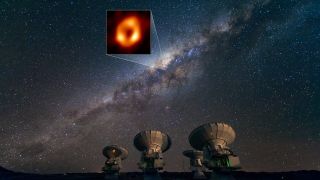
Famous submilimeter telescopes
Because submillimeter waves get absorbed by water in Earth's atmosphere, observatories that study sources of submillimeter radiation in the universe need to be built in high and dry places to prevent water vapor from obscuring their views. In essence, you will find submillimeter telescopes in the same places on Earth where you find the best optical telescopes.
The Atacama Large Millimeter/submillimeter Array (ALMA) , operated by the European Southern Observatory is located on the Chajnantor plateau in northern Chile at an altitude of 16,400 feet (5,000 m).
The Submillimeter Array on Hawai'i's Maunakea, which is operated by the Harvard Smithsonian Center for Astrophysics, sits somewhat lower, at 13,450 feet (4,100 m) above sea level.
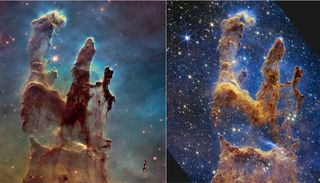
What does infrared light teach us about the universe?
Unlike submillimeter waves, infrared light spans a vast range of the electromagnetic spectrum from 0.04 inches (just below 1 millimeter) on the side bordering with microwaves to 0.75 micrometers on the side bordering with the visible light.
The NASA-led James Webb Space Telescope (JWST), launched on Christmas Day, 2021, thrust infrared astronomy into the spotlight with its ability to see the farthest reaches of the universe.
Infrared light, which is essentially heat, was the first non-visible wavelength discovered, completely by accident, by British astronomer William Herschel in 1800 during his experiments with the visible light spectrum. It took, however, a long time for infrared detectors to become sensitive enough to provide the breathtaking views of the cosmos that JWST is now known for.
The first crude observations of celestial objects in the infrared spectrum focused on the moon and the sun . Astronomers in the second half of the 19th century were able to measure the temperature of the sun 's atmosphere as well as the various temperature zones on the moon's surface. By the turn of the century, technology progressed to the level that it was possible to detect heat from the solar system's giant planets Jupiter and Saturn , according to A brief history of infrared astronomy .
Infrared astronomy, however, didn't fully take off until the second half of the 20th century when more sophisticated detectors were developed, allowing astronomers to analyze heat sources across the Milky Way.
Related: James Webb Space Telescope images: 12 astonishing views of our universe (gallery
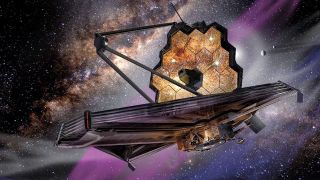
As the JWST has plentifully demonstrated since the release of its first images in July, 2022, infrared light is good at many things.
Thanks to its ability to penetrate through dust and gas, infrared light reveals what's going on inside of thick dust and gas clouds where stars form. Stars emerging in the middle of these clouds are not yet hot enough to emit visible light, but are warm enough to be detected by infrared sensors.
With such advanced technology as the JWST, astronomers can observe matter that is only several degrees warmer than absolute zero, the temperature of minus 459.67 degrees Fahrenheit (minus 273.15 degrees Fahrenheit), where the motion of atoms stops.
When viewing the Milky Way in infrared light, a hidden galaxy of failed stars, called brown dwarfs, emerges. Brown dwarfs are bodies that are too big to be called planets but are not quite massive enough to ignite nuclear fusion in their cores. Bodies in the farther reaches of the solar system that receive too little solar illumination also spring into view. Even the interstellar medium , the cool gas and dust dispersed between stars and galaxies, can be mapped in the infrared spectrum.
Webb was built with the aim to detect the first light that lit up the universe a few hundred million years after the Big Bang. Although this light had been emitted in the optical wavelength range, the accelerating expansion of the universe had stretched this light into the infrared range thanks to the effect known as redshift. Optical telescopes, even if they were as sensitive as Webb, could therefore no longer see this light.
But the James Webb Space Telescope sees only a small fraction of the infrared spectrum, the so-called mid and near-infrared light, which spans wavelengths from 28.5 micrometers to 0.6 micrometers where the visible spectrum begins.
NASA's recently retired flying telescope SOFIA was a specialist in the longer wavelength type of infrared light, the so-called far infrared, which reaches all the way to 612 micrometers and is best for observing the cool interstellar medium.
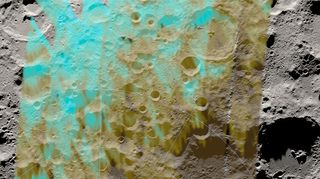
Famous infrared telescopes
Both, the James Webb Space Telescope and SOFIA, the current and recently retired (respectively) infrared astronomy flagships, had their predecessor.
NASA's Spitzer Space Telescope surveyed the universe in the mid-infrared and parts of the far infrared spectrum from 2003 to 2020. ESA's Herschel spacecraft complemented this work in the far-infrared spectrum between 2009 and 2013.
An earlier airborne telescope, the Kuiper Airborne Observatory , studied the infrared sky from 1974 to 1995.
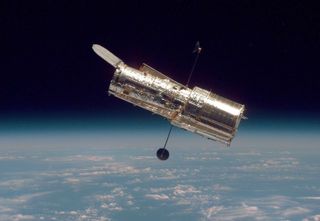
What does optical light teach us about the universe?
Optical astronomy has made enormous leaps since those first early 17th-century telescopes. Enhancing the natural abilities of the human eye beyond imagination, 21st-century optical telescopes are still the backbone of astronomy research.
From giant telescopes occupying remote mountain tops and highland plateaus to orbiting super-eyes such as the iconic Hubble Space Telescope , optical observatories reveal the universe with an ever-increasing level of detail. Some, on the other hand, focus on scanning vast swaths of the sky at once to spot unexpected phenomena, such as supernova explosions of dying stars or approaching asteroids .
Optical telescopes show the universe as it would appear to human eyes. Colors in optical images correspond to the colors human eyes would see. Images from other types of telescopes, such as those imaging the universe in infrared and ultraviolet light, have to be processed by astronomers on the ground, with colors artificially assigned to different wavelengths.
To be visible in the optical wavelengths, objects need to either emit their own visible light or be illuminated by other objects. Planets, moons and asteroids in our solar system are only visible to optical telescopes (and to human eyes) because of the vicinity of our sun.
Optical light can't pass through obstacles, such as thick clouds of dust, which hide some of the most interesting areas of the universe (such as centers of galaxies where supermassive black holes devour huge amounts of material or star-forming nebulas).
Optical light is also somewhat affected by Earth's atmosphere, even though not as much as the infrared and submillimeter wavelengths. While infrared and submillimeter radiation gets mostly absorbed, optical rays get a little dispersed by the molecules in the atmosphere, which means that observed objects don't appear as sharp as they would if the atmosphere wasn't present. This atmospheric blurring limits the accuracy of observations that Earth-based optical telescopes can achieve, even though modern adaptive optics systems installed on the world's best telescopes can to a certain extent make up for this shortcoming.
Aside from complex, costly machines in space and on remote mountain tops, optical astronomy is the most accessible method of observing the sky for amateur skywatchers. Decent backyard telescopes can be purchased for a few hundred dollars and Space.com provides plenty of guides on how to pick the best one for you.
Related: Best telescopes for seeing planets in 2023
Best telescopes 2023: For stargazing galaxies, nebulas and more
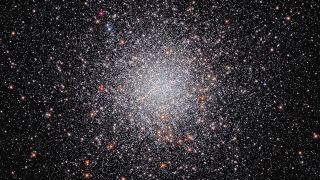
Famous optical telescopes
The Hubble Space Telescope is the undisputed king of optical astronomy and the source of many images that have gained iconic status. The telescope, launched in 1990, is still going strong and still may have a decade or so of life and fabulous astronomy ahead of it.
The Very Large Telescope (VLT) operated by the European Southern Observatory (ESO) in Chile is one of the most advanced Earth-based optical telescopes. VLT consists of four main telescopes, each with a 27-foot-wide (8.2 meter) mirror, and four 5.9-foot-wide (1.8 m) auxiliary telescopes. The four main telescopes can each detect light that is four billion times fainter than what human eyes can see. The telescopes can also work together as a so-called interferometer , which increases the resolution to a level that would be achievable with a single telescope with a 426-foot-wide (130 m) mirror.
ESO is currently building the next-generation Extremely Large Telescope (ELT), also in Chile. With a single 130-foot-wide (39.3 m) mirror , ELT will be the world's largest optical telescope. Once completed, the observatory will be able to gather 100 million times more light than the human eye and provide images 16 times sharper than the Hubble Space Telescope, according to ESO.
The twin Keck Telescopes on the Hawaiian island of Maunakea are fitted with 32.8-foot-wide (10 m wide) mirrors that forced the technical teams that designed and built them in the late 1980s to develop some ingenious technical solutions. Since it wasn't possible at that time to accurately operate a single solid mirror of such a size, engineers made the Keck mirrors from 36 hexagonal segments that work together as a unit with the help of an active optics system. This segmented mirror design is quite similar to the one used for the 21-foot-wide (6.5 m) mirror of the James Webb Space Telescope.
The Large Binocular Telescope in Arizona features the world's largest non-segmented mirror, measuring 28 feet (8.4 m) in diameter.
The Gran Telescopio Canarias on the Spanish island of La Palma off the coast of western Africa, is the world's largest single-aperture optical telescope, featuring a 10.4 m wide mirror.
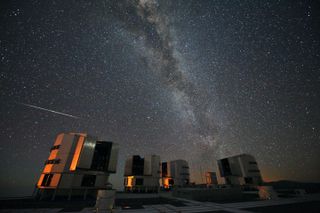
What does ultraviolet light teach us about the universe?
The great Hubble is also the world's main observer of ultraviolet light that emanates from sources in the universe. Ultraviolet light has shorter wavelengths and carries higher energies than visible light and points astronomers to hot, energetic processes, such as those taking place in young stars and in young star-forming galaxies . Massive stars that orbit each other in binary systems also emit ultraviolet light and so do powerful auroras on giant gaseous planets like Jupiter.
Ultraviolet light gets absorbed by the ozone layer in Earth's atmosphere, which is good for organisms living on Earth (as these wavelengths are known to cause tissue-damage and cancer). For astronomy, however, the limited ability of ultraviolet light to penetrate the atmospheres means that telescopes designed to study it need to orbit in space.
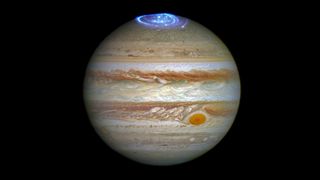
Famous ultraviolet telescopes
Apart from the Hubble Space Telescope, solar observatories such as the European Solar Orbiter or NASA's Solar Dynamics Observatory carry ultraviolet imagers to observe highly energetic processes on the sun. NASA's Jupiter explorer Juno also carries an instrument for studying ultraviolet light.
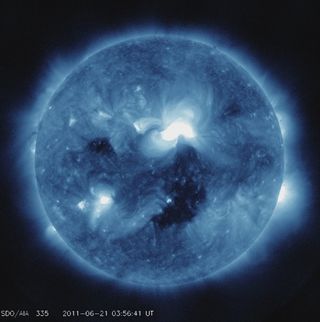
What do X-rays teach us about the universe?
Things get even more heated and energetic with X-rays. Discovered accidentally by German physicist Wilhelm Roentgen in 1895, these matter-penetrating rays are generated in vast amounts during some of the most extraordinary processes in the universe, such as when supermassive black holes or extremely massive neutron stars suck in matter from their surroundings, or during supernova explosions of dying stars.
X-rays come from the hottest places in the universe including black hole and neutron stars' accretion disks where matter spirals at extreme speeds. High-temperature plasma that fills space between galaxies in galaxy clusters also emits X-rays, and so do stars including our sun.
Astronomers recently discovered that comets can emit X-rays, Wibisono said, and that Jupiter, in addition to its ultraviolet aurora, also produces an aurora that shines in X-rays.
"X-rays are a really powerful part of the spectrum because you get fluorescence in X-rays," said Wibisono. "Rocky surfaces of moons and planets give off X-rays for fluorescence. The atmospheres around terrestrial planets also fluoresce and X-rays, the gas giants scatter solar X-rays, so they act like a mirror to the solar X-rays."
Fluorescence is the ability of a surface to absorb and subsequently emit light that originally arrived from another source.
Infamous for their potential to cause DNA mutations that may lead to cancer, X-rays get, just like ultraviolet rays, fortunately filtered out by Earth's atmosphere. X-ray astronomy could therefore only take off once humans were able to send objects to space. Astronomers knew prior to that that the sun is a powerful source of X-rays, but the first instruments capable of detecting other sources of cosmic X-rays were only launched aboard sounding rockets in the 1960s.
One of the problems with the detection of cosmic X-rays is their ability to penetrate matter. Just like they penetrate human tissue to reveal broken bones, X-rays also pass through mirrors that astronomers may want to use to concentrate them.
Building sensitive X-ray detectors therefore requires some engineering ingenuity. Scientists have to design mirrors for X-ray telescopes in a way that the energetic rays hit the reflecting surface at a shallow angle "like a stone skipping across the surface of a pond," according to NASA .
X-ray telescopes require multiple mirrors positioned at gradually increasing angles to deflect the X-rays onto a detector. Such contraptions, however, tend to be rather chunky and require large satellites to accommodate them. NASA's Chandra, for example, at 45-feet-long (13 m), is the largest satellite launched by the Space Shuttle , about a three feet (1 m) longer than Hubble.
The matter-penetrating ability of X-rays, however, also has its advantages, as these rays easily escape from dust-shrouded regions, such as galactic centers where black holes munch on the infalling matter.
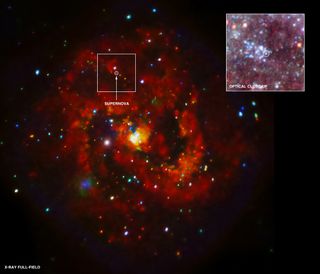
Famous X-ray telescopes
NASA's Chandra X-ray observatory is the current flagship X-ray telescope. In space since 1999, Chandra travels around Earth on an elliptical orbit that takes it as far as 83,000 miles (133,000 km) away from the planet's surface where no residual atmosphere obstructs the X-ray views. During its more than two decades in orbit, Chandra has imaged jets of matter shooting from supermassive black holes in galactic centers and even traced the separation of dark matter from normal matter in the collisions of galaxies in galactic clusters.
ESA also has its X-ray space observer, the XMM-Newton space telescope , also launched in 1999.
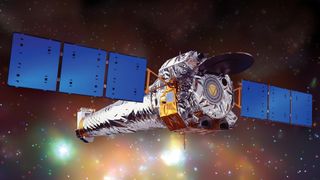
What do gamma-rays teach us about the universe?
— The 10 biggest telescopes on Earth — James Webb Space Telescope's 1st year in space has blown astronomers away — Best telescopes for kids 2023: Astronomy for all the family
Gamma-rays are the highest energy type of radiation present in the universe. Just like X-rays, they come from extremely hot and energetic processes in the universe, such as supernova explosions and accreting black holes. Even more capable of penetrating matter than X-rays, gamma-rays are also produced during nuclear explosions on Earth, and, in smaller quantities, in thunderstorms and during radioactive decay. Stars such as our sun also produce occasional gamma-ray flashes in the form of solar flares .
Just like many other types of astronomy, gamma-ray astronomy came about by accident. In the 1960s, American military satellites were looking for signs of the USSR's testing of nuclear weapons, when they detected inexplicable flashes of extremely energetic gamma-rays. Lasting from fractions of seconds to several minutes, these gamma-ray bursts , as they became known, were coming regularly from all parts of the universe.
It took until the 1990s for astronomers to figure out that these bursts come from extremely powerful explosions that mark the birth of new black holes when massive stars die. The shorter types of gamma-ray bursts are produced in collisions of superdense stellar remnants called the neutron stars.
Gamma-ray bursts point astronomers to the fact that a cataclysmic event has just occurred somewhere in the universe. By measuring the intensity of the burst, astronomers can learn something about the intensity and distance of the event. However, they need to search for the source of the flash afterward, using other types of telescopes. When they manage to locate the region in the sky where the burst has come from, they can then observe the area in other parts of the electromagnetic spectrum to gain more insight into the processes involved.
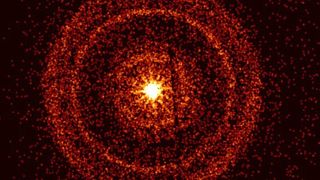
Famous gamma-ray telescopes
NASA's space telescopes Fermi and Swift together with ESA's Integral are the world's current gamma-ray burst spotting workhorses. However, only Swift, which covers about 9% of the sky, has the ability to locate sources of these giant explosions.
Astronomers are therefore looking for new approaches to gamma-ray burst detection. In 2021, a team of scientists from Hungary and Slovakia launched a tiny cubesat called GRB Alpha , that has been successfully detecting gamma-ray bursts ever since. In October 2022, GRBAlpha made an accurate detection of the peak intensity of the brightest gamma-ray burst ever seen, while the event completely blinded detectors on NASA's Fermi.
The researchers envision that a fleet of such cubesats would make it possible to find sources of gamma-ray bursts across the entire sky through the so-called triangulation, the same method used to pinpoint a location on Earth with the help of GPS .
Follow Tereza Pultarova on Twitter @TerezaPultarova . Follow us on Twitter @Spacedotcom and on Facebook .
Astronomy Cast, produced by the Planetary Science Institute in collaboration with Universe Today has a great series on the different kinds of astronomy based on the electromagnetic spectrum. You can listen to their radio astronomy , submillimeter astronomy , infrared astronomy , optical astronomy , ultraviolet astronomy , X-ray astronomy and gamma-ray astronomy .
National Radio Astronomy Observatory: The History of Radio Astronomy, accessed April 2023 from: https://public.nrao.edu/radio-astronomy/the-history-of-radio-astronomy/
Walker, J. H. A brief history of infrared astronomy, Astronomy & Geophysics, Volume 41, Issue 5, October 2000, Pages 5.10–5.13: https://doi.org/10.1046/j.1468-4004.2000.41510.x
ESA: Seeing with infrared eyes: A brief history of infrared astronomy, July 2020, accessed April 2023 from: https://sci.esa.int/web/herschel/-/59550-a-brief-history-of-infrared-astronomy
Harvard University: History of X-Ray Astronomy, accessed April 2023 from: https://chandra.harvard.edu/xray_astro/history.html
ESA: History of X-ray astronomy in Europe: From Exosat to ATHENA, accessed April 2023 from: https://sci.esa.int/web/athena/-/60759-history-of-x-ray-astronomy-in-europe-from-exosat-to-athena
Pinkau, K. History of gamma-ray telescopes and astronomy, Experimental Astronomy, Volume 25, Issue 1-3, pp. 157-171, August 2009: https://ui.adsabs.harvard.edu/abs/2009ExA....25..157P/abstract
Join our Space Forums to keep talking space on the latest missions, night sky and more! And if you have a news tip, correction or comment, let us know at: [email protected].
Get the Space.com Newsletter
Breaking space news, the latest updates on rocket launches, skywatching events and more!
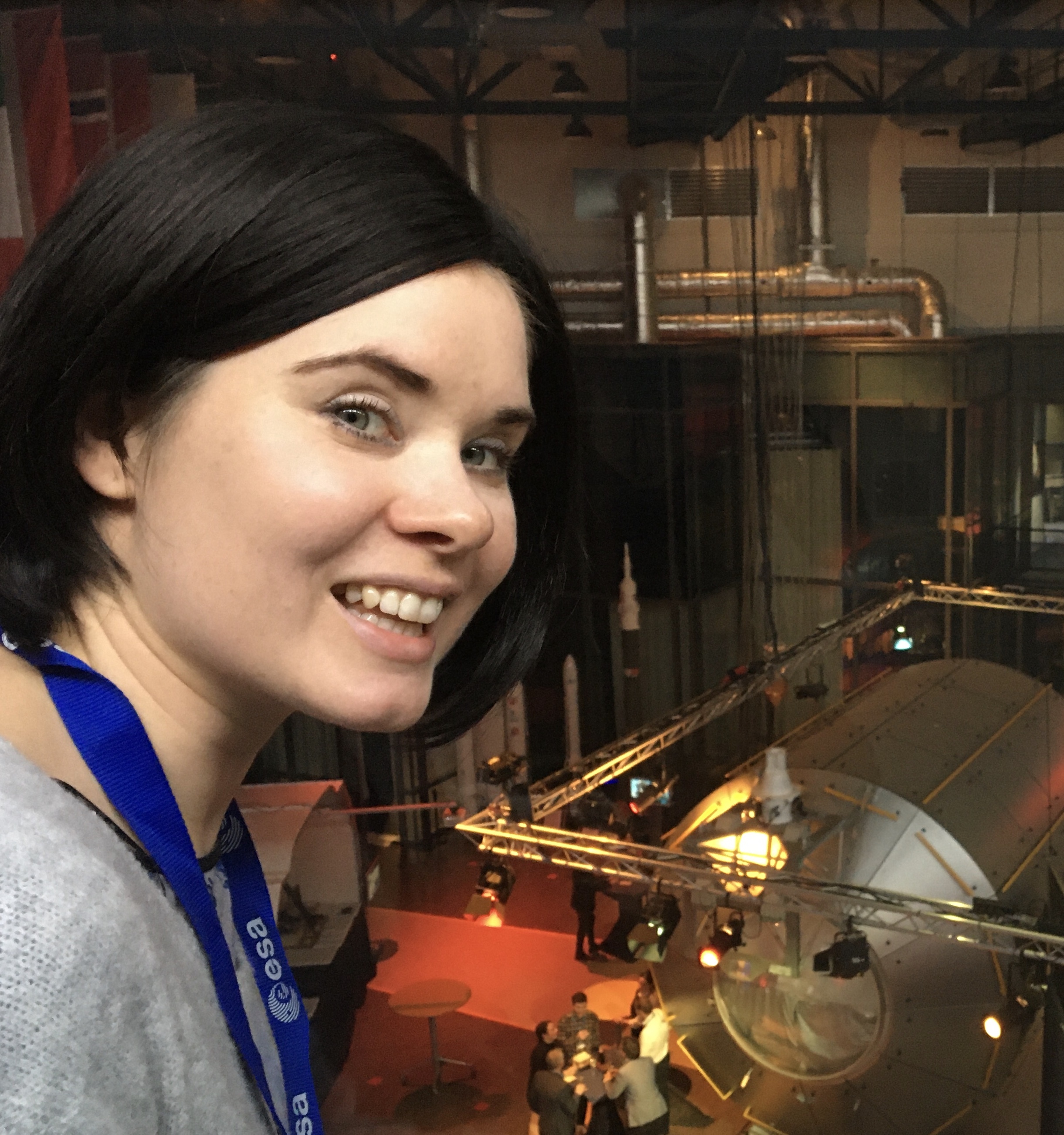
Tereza is a London-based science and technology journalist, aspiring fiction writer and amateur gymnast. Originally from Prague, the Czech Republic, she spent the first seven years of her career working as a reporter, script-writer and presenter for various TV programmes of the Czech Public Service Television. She later took a career break to pursue further education and added a Master's in Science from the International Space University, France, to her Bachelor's in Journalism and Master's in Cultural Anthropology from Prague's Charles University. She worked as a reporter at the Engineering and Technology magazine, freelanced for a range of publications including Live Science, Space.com, Professional Engineering, Via Satellite and Space News and served as a maternity cover science editor at the European Space Agency.
Car-size asteroid gives Earth a super-close shave with flyby closer than some satellites
SpaceX launches advanced weather satellite for US Space Force (video)
ULA chronicles the rise of Vulcan rocket in new employee-drawn comic book
- rod How does BB explain the origin of the electromagnetic spectrum? For example, why should there be optical light vs. nothing to see at all? Consider some past discussions on issues like this in science. https://forums.space.com/threads/one-of-the-greatest-damn-mysteries-of-physics-we-studied-distant-suns-in-the-most-precise-astronomical-test-of-electromagnetism-yet.59494/ https://forums.space.com/threads/how-do-we-know-the-fundamental-constants-are-constant-we-dont.59359/ https://forums.space.com/threads/what-makes-newtons-laws-work-heres-the-simple-trick.57302/ Reply
- Pentcho Valev The underlying assumptions are that the universe is expanding and that the speed of light is constant. However the expanding-universe theory is too preposterous and will soon be abandoned. Then the only reason behind the cosmological (Hubble) redshift will turn out to be GRADUALLY DECREASING SPEED OF LIGHT. And cosmologists will have to start from the scratch. Reply
- murgatroyd Good overview article, thanks! Reply
- rod "This uniformness is unseen in other wavelengths, which reveal the sky in dots and regions of varying brightness. In fact, cosmic microwave radiation is so odd that the researchers who first discovered it in the 1960s (completely by accident during experiments with echo balloons) originally thought it was produced by a telescope defect. Subsequent research, however, confirmed that the microwave hum was coming from space and that it was nothing less than the residue of radiation released by the Big Bang, the enormous explosion which created the universe some 13.8 billion years ago." According to cosmology calculators, depending upon the input value for H0, the universe radius when the CMBR became light was about 40-41 million light years radius. In the spectrum of the CMBR, where is the H-alpha line and He showing the universe then was filled with H and He gas? The same applies to the H1 21-cm line for H gas filling the universe during the cosmic dark ages and showing zero-metal gas? Reply
rod said: According to cosmology calculators, depending upon the input value for H0, the universe radius when the CMBR became light was about 40-41 million light years radius. In the spectrum of the CMBR, where is the H-alpha line and He showing the universe then was filled with H and He gas? The same applies to the H1 21-cm line for H gas filling the universe during the cosmic dark ages and showing zero-metal gas?
Helio said: If I understand it, the photons were highly scattered during those 40 million years until the universe cooled, due to expansion, to about 3000K (2.73 x 1100). Suddenly, the electrons became bound to the protons, forming the first atoms. Thus, all those photons were released to go flying in every direction without all that scattering. So we’re not seeing emissions from h or he, hence no emission or absorption lines.
rod said: However, Helio, others do not see this as you present. Hydrogen absorption lines in the cosmic microwave background spectrum, https://www.researchgate.net/publication/226565853_Hydrogen_absorption_lines_in_the_cosmic_microwave_background_spectrum, August 2004. "We have calculated the intensities of the subordinate hydrogen lines formed during the recombination epoch at redshifts 800≲z≲1600. We show that an allowance for the angular momentum splitting of hydrogen atomic energy levels and the dipole transition selection rules can reveal absorption features in the cosmic microwave background recombination spectrum in the submillimeter wavelength range." Impact of inhomogeneous CMB heating of gas on the HI 21-cm signal during dark ages, https://arxiv.org/abs/1810.05908
Admin said: Frequencies of light invisible to the human eye reveal a vast amount of information about our universe. But it took decades for scientists to learn how How does astronomy use the electromagnetic spectrum? : Read more
rod said: However, Helio, others do not see this as you present. Hydrogen absorption lines in the cosmic microwave background spectrum, https://www.researchgate.net/publication/226565853_Hydrogen_absorption_lines_in_the_cosmic_microwave_background_spectrum,
Helio said: In their short conclusion, they seem to confirm the spectral distribution that would exist for the Recombination event. Recombination, of course, is a major prediction of the BBT, giving us the CMBR. That they can see some Hβ and Hγ lines is interesting, and not contrary to BBT.
- View All 21 Comments
Most Popular
- 2 Watch an exclusive clip from the CNN' 'Space Shuttle Columbia: The Final Flight' finale (video)
- 3 'Fly Me to the Moon' trailer mixes real-life Apollo history with moon landing hoax
- 4 HALO Space unveils capsule design for stratospheric space 'glamping'
- 5 One of the universe's most 'extreme' dead stars just sprang back to life unexpectedly
- Anatomy & Physiology
- Astrophysics
- Earth Science
- Environmental Science
- Organic Chemistry
- Precalculus
- Trigonometry
- English Grammar
- U.S. History
- World History
... and beyond
- Socratic Meta
- Featured Answers

How do electromagnetic waves travel?

See the following pages for more information: http://www.pion.cz/en/article/electromagnetic-spectrum
Related questions
- What causes gamma ray bursts?
- How are gamma ray bursts classified?
- How do gamma ray bursts affect earth?
- How far do gamma ray bursts travel?
- When the ultraviolet light from hot stars in very distant galaxies finally reaches us, it...
- What does ultraviolet light from hot stars in very distant galaxies reaches us in the form of?
- What are some advantages and disadvantages for using an ultraviolet telescope? Why is at...
- How is imaging spectroscopy used in astronomy?
- What is spectroscopy? How does it detect the electromagnetic spectrum?
- What techniques are used to study the electromagnetic spectrum in space?
Impact of this question

StarsInsider
What is electromagnetic radiation, and how concerned should we be?
Posted: April 13, 2024 | Last updated: April 13, 2024
What is electromagnetic radiation, and how wary should we be?
The word "radiation" carries some understandably worrisome connotations. From harmful radioactive waste to apocalyptic nuclear catastrophes , electromagnetic radiation is certainly something to be cautious about. However, that type of radiation is only one far end of the spectrum. On the other end are visible light waves and radio waves that we come into contact with and even depend upon every day. In order to be properly prepared to deal with electromagnetic radiation in a safe way, it's important to get the full picture.
So, what exactly are electromagnetic rays, how does their radiation affect us, how do we use it, and how can it hurt us? Read on to find out.
You may also like: Rock of ages: The world's biggest music stars, then and now
Electromagnetic waves
Electromagnetic waves might not be seen, but they're all around us and have a hand in countless aspects of life that we might take for granted. Certain types of electromagnetic waves, however, in certain quantities, can be dangerous.
Follow us and access great exclusive content every day
A short history
Electromagnetic waves weren't invented, but discovered and then harnessed. They've been around forever, since the birth of the universe . It wasn't until the 19th century, however, that their existence was proven.
You may also like: Royals caught off-guard
Heinrich Hertz
Heinrich Hertz, born in Germany in 1857, was the first person to definitively prove the existence of electromagnetic waves. He experimented with putting their power to specialized use, sending these waves from one antenna to another.
The electromagnetic spectrum
Since the days of Hertz, we have gained a far stronger understanding of the world of electromagnetic waves. The nature and effects of these waves change with the shapes of their wavelengths.
You may also like: The world's ugliest (but coolest) dogs
Visible light
Visible light does in fact travel along electromagnetic waves. In relation to the electromagnetic spectrum, visible light and the colors perceivable by the human eye travel on wavelengths between 380 to 700 nanometers in length.
Ultraviolet rays
Ultraviolet rays, which aren't visible to the naked eye, are ever so slightly shorter than visible light waves, resting between 400 nanometers and 10 nanometers. Some insects, such as bees, can detected ultraviolet light, and astronomers can use UV rays to observe otherwise invisible happenings in the cosmos.
You may also like: The world's most well-traveled citizens
Radio waves
Radio waves are the longest and largest wavelengths on the electromagnetic spectrum, with arcs that can be as tall as a 10-story building, but are still imperceptible to the human eye. Radio waves can be detected bouncing all across not only the planet, but the galaxy and the universe at large.
Microwaves are one of the most popular and well-known electromagnetic waves, ever since their convenient cooking capabilities were widely utilized in the 1970s. They're also used by meteorologists around the world to track weather movements.
You may also like: Celebrity siblings that fame forgot
Infrared waves can't be seen, but can be felt as heat by humans. This is why infrared waves are utilized in heat imaging. We also have infrared waves to thank for our convenient television remotes.
Despite being some of the most dangerous waves on the electromagnetic spectrum, they are also utilized to do the most good. X-rays have some of the shortest wavelengths on the spectrum, meaning they can penetrate lots of solid material such as human skin, but aren't quite small enough to penetrate bone. This is why X-rays can be used by the medical community to easily look inside the body and check for bone injuries and tumors.
You may also like: What are the most common star signs of serial killers? You won't guess the answer
Gamma rays inhabit the far end of the electromagnetic spectrum, and are the shortest of the wavelengths. Their size and concentration verges on incomprehensible, measuring in at mere tenths of an angstrom, a unit of measurement equal to 0.0000000001 meter. This size enables gamma rays to penetrate just about any material on earth, and is one of the strongest electromagnetic powers in the universe. Gamma rays are produced by only the most intense energy expulsions in the universe, such as nuclear explosions and supernovas.
Electromagnetic radiation
Simply put, radiation is just the movement of electromagnetic waves through any given space or material. Thus, radiation isn't inherently damaging. The largest electromagnetic waves, like massive radio waves and visible light waves, have no way of penetrating any type of human tissue and therefore pose no real danger. But smaller and more energetic waves like X-rays and gamma rays have more penetration power, and are extremely harmful to humans and other biological life.
You may also like: Nicole Kidman's transformations on and off-screen
An invisible world all around us
Electromagnetic waves in all their forms are all around us, and in fact stretch across the entire known universe. We may not see most of them, but they all clearly affect our lives in vastly different ways.
No need for molecules
What makes electromagnetic waves so powerful is their freedom of movement. They don't rely on molecules or solid matter to move around, and can thus move through thin air and space at will.
You may also like: Are these celebs better blonde or brunette?
The benefits of electromagnetic waves
Many electromagnetic waves, especially on the larger side of the spectrum, can be harnessed in ways that are greatly beneficial to us.
The family microwave
The most obvious example is, of course, the aforementioned microwave. Ever since the 1970s, these wonderful boxes that emit, well, microwaves, have made rushed meals more convenient than ever.
You may also like: Actors who got into directing
Getting your tan on
Ultraviolet rays can be harmful and even cancerous in excessive amounts, but they're also to thank for our deep summer tans. Just make sure to wear your sunscreen!
The wireless world
Every wireless phone call, internet connection, and file transfer rides along electromagnetic wavelengths. We can all agree that life without these luxuries is almost unimaginable!
You may also like: Celebs who are expecting or had babies in 2020
What makes certain electromagnetic waves dangerous?
As we have seen, electromagnetic waves can also cause serious damage. Extremely powerful waves like gamma rays can cause immediate damage, but prolonged exposure to other waves like ultraviolet and infrared can also be harmful.
The dangers of electromagnetic waves
The dangers associated with electromagnetic waves are just as wide and varied as the wavelengths themselves, and can range from minor annoyances to death sentences.
You may also like: History's most notorious lies, hoaxes, and deceptions
Damage inside and out
Electromagnetic radiation can cause minor surface damage, such as sunburns or retina damage, but can also produce the kind of catastrophic and horrifying destruction seen in tragedies like Hiroshima and Nagasaki and Chernobyl.
The threat of cancer
While gamma rays released in nuclear explosions are so destructive that cancer doesn't even have a chance to develop, other rays like the ultraviolet waves that are emitted from the sun can cause cancer if proper precautions aren't taken.
You may also like: Hacks to keep food fresh for longer
How to protect from electromagnetic radiation
Thankfully, there are many ways to reduce exposure to potentially harmful electromagnetic radiation that are easy and affordable.
Keep the router away
Wi-fi routers are in almost every home and office building around the world, and are so ubiquitous that their presence is often forgotten. While only nominally harmful, some experts say putting your router in a less frequently trafficked part of your house can help minimize exposure to radio waves.
You may also like: These celebrities were banned from famous talk shows
Use speaker phone
The same goes with cell phones. Using speaker phone keeps your radio wave-emitting device further from your ears and brain, where they might do damage over long periods of time.
Keep the shades and sunscreen on
Ultraviolet rays are best combated by a good pair of sunglasses and a healthy coating of sunscreen. To avoid the chances of developing an eye or skin condition, make sure you always have these tools handy when you head to the beach.
You may also like: This stunning Brazilian island has a terrifying backstory
Rewire the bedroom
Bedrooms are more and more epicenters of wireless activity. From cell phones to smart bulbs and Bluetooth speakers, there are often numerous electromagnetic waves bouncing all around at the same time. You can practice good bedroom hygiene by going back to the basics: use wired speakers, keep your router somewhere else, and turn your phone off when it's time to go to sleep.
Airplane mode
Your smartphone's airplane mode doesn't just have to be for flights. You can also use airplane mode to minimize electromagnetic radiation from your phone by using airplane mode whenever you're not actively using it or expecting an important call.
You may also like: What really happens during an autopsy
The 5G controversy
A few years ago, the advent of 5G broadband for cell networks caused quite a stir, with some critics claiming that the electromagnetic radiation emitted from 5G towers was stronger and more dangerous than anything seen before. According to the World Health Organization, however, the radiation emitted from 5G-capable devices is "negligible."
Sources: (Britannica) (NASA) (World Health Organization)
See also: Surprisingly radioactive things you have at home
More for You
Peanuts by Charles Schulz
Masters 2024: Low amateur's press conference ends with super awkward exchange regarding Tiger Woods
The Only 2 Ingredients You Need For Luscious Fudge Cookies
Top 10 Times Bill Hader Broke The SNL Cast
Tax Day deals 2024: Score discounts, freebies at Krispy Kreme, Hooters, Potbelly, more
How many of the 50 best movies of the '60s have you seen?
Indiana Fever picks first in star-studded WNBA draft with Caitlin Clark. See full draft order
Disney Cites First Amendment In Gina Carano Termination Suit
What It Means If Your Costco Rotisserie Chicken Is Green
New 'Beetlejuice Beetlejuice' Footage Introduces Jenna Ortega's New Character
The 43 Best Shows to Stream on Netflix Right Now
The Worst Plane Seats You Should Never Pick When Flying
"He basically bought out everybody else; he wouldn't buy me out" - Nelly on being Bobcats co-owner with Michael Jordan
I downsized from my city apartment to a tiny house in the countryside to save money – but my cost of living increased instead
15 Boxed Cake Flavors That Are Probably Gone Forever
Fallout from Trump's bid to overturn election loss heads to Supreme Court
10 Actors Who Got Fired From Roles Of A Lifetime
‘Mr. Monk's Last Case: A Monk Movie' Might Not Be His Last Case After All, Tony Shalhoub Says – Contenders TV
13 Polite Habits Flight Attendants Actually Dislike—and What to Do Instead
The Cavs threw last game to manipulate NBA Playoff matchups, and it could haunt them
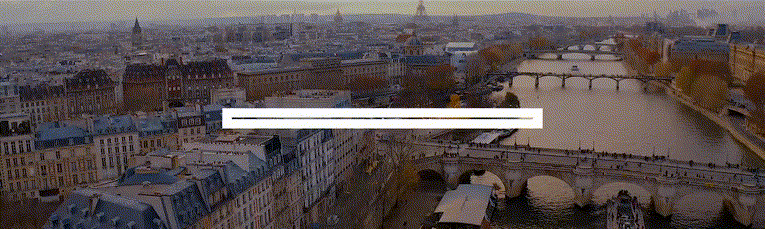
IMAGES
VIDEO
COMMENTS
This means that electromagnetic waves can travel not only through air and solid materials, but also through the vacuum of space. In the 1860's and 1870's, a Scottish scientist named James Clerk Maxwell developed a scientific theory to explain electromagnetic waves. He noticed that electrical fields and magnetic fields can couple together to ...
Maxwell's correction shows that self-sustaining electromagnetic waves (light) can travel through empty space even in the absence of moving charges or currents, with the electric field component and magnetic field component each continually changing and each perpetuating the other. ... Electromagnetic Wave: Electromagnetic waves are a self ...
Electromagnetic radiation is one of the many ways that energy travels through space. The heat from a burning fire, the light from the sun, the X-rays used by your doctor, as well as the energy used to cook food in a microwave are all forms of electromagnetic radiation. While these forms of energy might seem quite different from one another ...
Electromagnetic radiation can travel through empty space. Most other types of waves must travel through some sort of substance. For example, sound waves need either a gas, solid, or liquid to pass through in order to be heard; The speed of light is always a constant (3 x 10^8 m/s)
Electromagnetic radiation is a form of energy that can travel through space and matter. It includes visible light, radio waves, microwaves, infrared, ultraviolet, X-rays and gamma rays. Learn more about the properties, sources, effects and applications of electromagnetic radiation from this Wikipedia article.
The Mechanism of Electromagnetic Wave Propagation. To see how the symmetry introduced by Maxwell accounts for the existence of combined electric and magnetic waves that propagate through space, imagine a time-varying magnetic field \(\vec{B}_0(t)\) produced by the high-frequency alternating current seen in Figure \(\PageIndex{3}\).
Mechanical waves travel through a medium such as a string, water, or air. Perhaps the most significant prediction of Maxwell's equations is the existence of combined electric and magnetic (or electromagnetic) fields that propagate through space as electromagnetic waves.
An electromagnetic wave begins when an electrically charged particle vibrates. This causes a vibrating electric field, which in turn creates a vibrating magnetic field. The two vibrating fields together form an electromagnetic wave. An electromagnetic wave is a transverse wave that can travel across space as well as through matter.
Electromagnetic waves travel through empty space at the speed of light in free space, c = 2.998 ⋅ 108m s, and through other materials at speeds less than c. For a sinusoidal electromagnetic wave, the speed of propagation is the product of the frequency and wavelength | →v | = fλ. where | →v | is the magnitude of the velocity in m s, f is ...
The speed of any electromagnetic waves in free space is the speed of light c = 3*10 8 m/s. Electromagnetic waves can have any wavelength λ or frequency f as long as λf = c. When electromagnetic waves travel through a medium, the speed of the waves in the medium is v = c/n(λ free), where n(λ free) is the
And the speed at which these waves travel is the speed of light, c, and by c I mean three times 10 to the eight meters per second, because light is just and Electromagnetic wave, light is a special example, one particular example of Electromagnetic waves, but it is only one example, these waves can have any wavelength.
Propagation of an Electromagnetic Wave. Electromagnetic waves are waves which can travel through the vacuum of outer space. Mechanical waves, unlike electromagnetic waves, require the presence of a material medium in order to transport their energy from one location to another. Sound waves are examples of mechanical waves while light waves are ...
Ergo, light is made of electromagnetic waves and it travels at that speed, because that is exactly how quickly waves of electricity and magnetism travel through space. And this was all well and ...
Electromagnetic radiation is, classically speaking, a wave of electric and magnetic fields propagating at the speed of light c through empty space. In this wave the electric and magnetic fields change their magnitude and direction each second. This rate of change is the frequency ν measured in cycles per second—namely, in hertz.
Examples of electromagnetic waves traveling through space independent of matter are radio and television waves, microwaves, infrared rays, visible light, ultraviolet light, X-rays, and gamma rays. All of these waves travel at the same speed—namely, the velocity of light (roughly 300,000 kilometres, or 186,000 miles, per second).
Electromagnetic waves are nothing but electric and magnetic fields travelling through free space with the speed of light c. An accelerating charged particle is when the charged particle oscillates about an equilibrium position. ... The wavelength λ of this wave is given by λ = c/f. Electromagnetic waves transfer energy through space ...
0. Since, electro magnetic waves have electric and magnetic vector. Due to this EM waves show electric and magnetic field. An electric and magnetic field have no need a medium to show thier effect. Hence in the presence of electric and magnetic field vector which vibrate perpendeculer to each other and get pertervation EM waves travels in vacuum.
Unlike sound waves, which must travel through matter by bumping molecules into each other like dominoes (and thus can not travel through a vacuum like space), electromagnetic waves do not need molecules to travel. They can travel through air, solid objects, and even space, making them very useful for a lot of technologies. ...
These are called mechanical waves . Sound waves, water waves, and seismic waves are all types of mechanical waves. Other waves, called electromagnetic waves can travel through a medium or through a vacuum where there is no matter, such as outer space. Light is a form of electromagnetic wave. The amplitude and frequency of both mechanical and ...
What do microwaves teach us about the universe? The next electromagnetic spectrum band after radio waves are microwaves. As microwaves cover wavelengths between 3.3 feet and 0.04 inches (1 meter ...
Electromagnetic waves propagate through space as varying magnetic and electrical fields (hence, 'electromagnetic'). There are two main differences between sound waves and light waves. The first difference is in velocity. Sound waves travel through air at the speed of approximately 1,100 feet per second; light waves travel through air and empty ...
This is not a contrived notion—it reflects how signals really travel across an interconnect and through real media. Signals travel through PCBs as electromagnetic waves, and the propagation behavior is determined in part by the wave impedance. ... experienced by an electromagnetic signal as it travels through free space (i.e., vacuum) or some ...
Visible light does in fact travel along electromagnetic waves. In relation to the electromagnetic spectrum, visible light and the colors perceivable by the human eye travel on wavelengths between ...